Parvulin 14 and Parvulin 17 Bind to HBx and cccDNA and Upregulate Hepatitis B Virus Replication from cccDNA to Virion in an HBx-Dependent Manner
- PMID: 30567987
- PMCID: PMC6401437
- DOI: 10.1128/JVI.01840-18
Parvulin 14 and Parvulin 17 Bind to HBx and cccDNA and Upregulate Hepatitis B Virus Replication from cccDNA to Virion in an HBx-Dependent Manner
Abstract
The parvulin 14 (Par14) and parvulin 17 (Par17) proteins, which are both encoded by the PIN4 gene, play roles in protein folding, chromatin remodeling, DNA binding, ribosome biogenesis, and cell cycle progression. However, the effects of Par14 and Par17 on viral replication have never been explored. In this study, we found that, in the presence of HBx, either Par14 or Par17 could upregulate hepatitis B virus (HBV) replication, whereas in the absence of HBx, neither Par14 nor Par17 had any effect on replication. Overexpression of Par14/Par17 markedly increased the formation of covalently closed circular DNA (cccDNA), synthesis of HBV RNA and DNA, and virion secretion. Conversely, PIN4 knockdown significantly decreased HBV replication in HBV-transfected and -infected cells. Coimmunoprecipitation revealed that Par14/Par17 engaged in direct physical interactions with HBx in the cytoplasm, nucleus, and mitochondria, possibly mediated through substrate-binding residues on Par14/Par17 (E46/D74 and E71/D99, respectively) and conserved 19R20P-28R29P motifs on HBx. Furthermore, these interactions enhanced HBx stability, promoted HBx translocation to the nuclear and mitochondrial fractions, and increased HBV replication. Chromatin immunoprecipitation assays revealed that, in the presence of HBx, Par14/Par17 were efficiently recruited to cccDNA and promoted transcriptional activation via specific DNA-binding residues (S19/44). In contrast, in the absence of HBx, Par14/Par17 bound cccDNA only at the basal level and did not promote transcriptional activation. Taken together, our results demonstrate that Par14 and Par17 upregulate HBV RNA transcription and DNA synthesis, thereby increasing the HBV cccDNA level, through formation of the cccDNA-Par14/17-HBx complex.IMPORTANCE The HBx protein plays an essential regulatory role in HBV replication. We found that substrate-binding residues on the human parvulin peptidylprolyl cis/trans isomerase proteins Par14 and Par17 bound to conserved arginine-proline (RP) motifs on HBx in the cytoplasm, nucleus, and mitochondria. The HBx-Par14/Par17 interaction stabilized HBx; promoted its translocation to the nucleus and mitochondria; and stimulated multiple steps of HBV replication, including cccDNA formation, HBV RNA and DNA synthesis, and virion secretion. In addition, in the presence of HBx, the Par14 and Par17 proteins bound to cccDNA and promoted its transcriptional activation. Our results suggest that inhibition or knockdown of Par14 and Par17 may represent a novel therapeutic option against HBV infection.
Keywords: HBV; HBV replication; HBx; cccDNA; parvulin 14; parvulin 17.
Copyright © 2019 American Society for Microbiology.
Figures
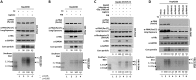
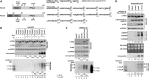
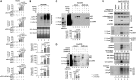
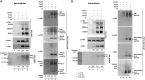
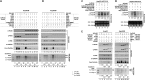
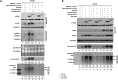
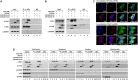
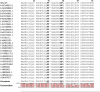
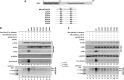
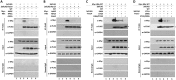
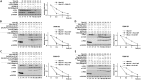
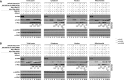
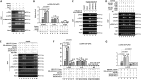
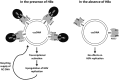
Similar articles
-
PPIases Par14/Par17 Affect HBV Replication in Multiple Ways.Viruses. 2023 Feb 6;15(2):457. doi: 10.3390/v15020457. Viruses. 2023. PMID: 36851672 Free PMC article. Review.
-
The HBV Core Protein and Core Particle Both Bind to the PPiase Par14 and Par17 to Enhance Their Stabilities and HBV Replication.Front Microbiol. 2021 Dec 14;12:795047. doi: 10.3389/fmicb.2021.795047. eCollection 2021. Front Microbiol. 2021. PMID: 34970249 Free PMC article.
-
Hepatitis B virus X protein counteracts high mobility group box 1 protein-mediated epigenetic silencing of covalently closed circular DNA.PLoS Pathog. 2022 Jun 9;18(6):e1010576. doi: 10.1371/journal.ppat.1010576. eCollection 2022 Jun. PLoS Pathog. 2022. PMID: 35679251 Free PMC article.
-
Hepatitis B virus X protein modulates remodelling of minichromosomes related to hepatitis B virus replication in HepG2 cells.Int J Mol Med. 2013 Jan;31(1):197-204. doi: 10.3892/ijmm.2012.1165. Epub 2012 Oct 26. Int J Mol Med. 2013. PMID: 23128981
-
Hepatitis B Virus Capsid: The Core in Productive Entry and Covalently Closed Circular DNA Formation.Viruses. 2023 Feb 28;15(3):642. doi: 10.3390/v15030642. Viruses. 2023. PMID: 36992351 Free PMC article. Review.
Cited by
-
Immunoinformatics and Evaluation of Peptide Vaccines Derived from Global Hepatitis B Viral HBx and HBc Proteins Critical for Covalently Closed Circular DNA Integrity.Microorganisms. 2023 Nov 21;11(12):2826. doi: 10.3390/microorganisms11122826. Microorganisms. 2023. PMID: 38137971 Free PMC article.
-
Ca2+/Calmodulin-Dependent Protein Kinase II Inhibits Hepatitis B Virus Replication from cccDNA via AMPK Activation and AKT/mTOR Suppression.Microorganisms. 2022 Feb 23;10(3):498. doi: 10.3390/microorganisms10030498. Microorganisms. 2022. PMID: 35336076 Free PMC article.
-
The cyclophilin inhibitor CRV431 inhibits liver HBV DNA and HBsAg in transgenic mice.PLoS One. 2019 Jun 10;14(6):e0217433. doi: 10.1371/journal.pone.0217433. eCollection 2019. PLoS One. 2019. PMID: 31181107 Free PMC article.
-
Canonical and Divergent N-Terminal HBx Isoform Proteins Unveiled: Characteristics and Roles during HBV Replication.Biomedicines. 2021 Nov 16;9(11):1701. doi: 10.3390/biomedicines9111701. Biomedicines. 2021. PMID: 34829930 Free PMC article.
-
The interaction of hepatitis B virus with the ubiquitin proteasome system in viral replication and associated pathogenesis.Virol J. 2019 May 30;16(1):73. doi: 10.1186/s12985-019-1183-z. Virol J. 2019. PMID: 31146743 Free PMC article. Review.
References
-
- World Health Organization. 2017. Global hepatitis report 2017. World Health Organization, Geneva, Switzerland. http://www.who.int/hepatitis/publications/global-hepatitis-report2017/en/. Accessed 20 August 2018.
-
- Yan H, Zhong G, Xu G, He W, Jing Z, Gao Z, Huang Y, Qi Y, Peng B, Wang H, Fu L, Song M, Chen P, Gao W, Ren B, Sun Y, Cai T, Feng X, Sui J, Li W. 2012. Sodium taurocholate cotransporting polypeptide is a functional receptor for human hepatitis B and D virus. Elife 1:e00049. doi:10.7554/eLife.00049. - DOI - PMC - PubMed
Publication types
MeSH terms
Substances
LinkOut - more resources
Full Text Sources
Medical
Molecular Biology Databases