Organotypic slice culture based on in ovo electroporation for chicken embryonic central nervous system
- PMID: 30565384
- PMCID: PMC6378233
- DOI: 10.1111/jcmm.14080
Organotypic slice culture based on in ovo electroporation for chicken embryonic central nervous system
Abstract
Organotypic slice culture is a living cell research technique which blends features of both in vivo and in vitro techniques. While organotypic brain slice culture techniques have been well established in rodents, there are few reports on the study of organotypic slice culture, especially of the central nervous system (CNS), in chicken embryos. We established a combined in ovo electroporation and organotypic slice culture method to study exogenous genes functions in the CNS during chicken embryo development. We performed in ovo electroporation in the spinal cord or optic tectum prior to slice culture. When embryonic development reached a specific stage, green fluorescent protein (GFP)-positive embryos were selected and fluorescent expression sites were cut under stereo fluorescence microscopy. Selected tissues were embedded in 4% agar. Tissues were sectioned on a vibratory microtome and 300 μm thick sections were mounted on a membrane of millicell cell culture insert. The insert was placed in a 30-mm culture dish and 1 ml of slice culture media was added. We show that during serum-free medium culture, the slice loses its original structure and propensity to be strictly regulated, which are the characteristics of the CNS. However, after adding serum, the histological structure of cultured-tissue slices was able to be well maintained and neuronal axons were significantly longer than that those of serum-free medium cultured-tissue slices. As the structure of a complete single neuron can be observed from a slice culture, this is a suitable way of studying single neuronal dynamics. As such, we present an effective method to study axon formation and migration of single neurons in vitro.
Keywords: central nervous system; chicken embryo; in ovo electroporation; optic tectum; organotypic slice culture; spinal cord.
© 2018 The Authors. Journal of Cellular and Molecular Medicine published by John Wiley & Sons Ltd and Foundation for Cellular and Molecular Medicine and John Wiley & Sons Ltd.
Figures
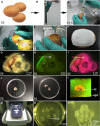
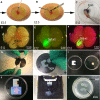
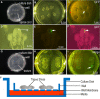
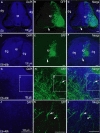
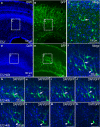
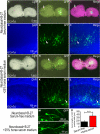
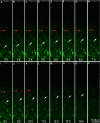
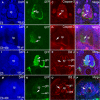
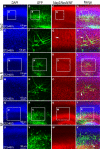
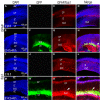
Similar articles
-
Combined use of in ovo electroporation and cultured neurons for gene function analysis of embryogenesis in the chicken optic tectum.Neuroreport. 2017 Dec 6;28(17):1180-1185. doi: 10.1097/WNR.0000000000000903. Neuroreport. 2017. PMID: 28953094
-
Use of in vitro electroporation and slice culture for gene function analysis in the mouse embryonic spinal cord.Mech Dev. 2019 Aug;158:103558. doi: 10.1016/j.mod.2019.103558. Epub 2019 Jun 15. Mech Dev. 2019. PMID: 31212004
-
In Ovo Electroporation of Embryonic Chicken Spinal Cord in Combination with Ex Vivo Slice Culture for Live Imaging of Vertebrate Neuronal Differentiation.Methods Mol Biol. 2024;2831:97-111. doi: 10.1007/978-1-0716-3969-6_8. Methods Mol Biol. 2024. PMID: 39134846
-
Cell death and proliferation in acute slices and organotypic cultures of mammalian CNS.Prog Neurobiol. 2009 Aug;88(4):221-45. doi: 10.1016/j.pneurobio.2009.01.002. Epub 2009 Jan 24. Prog Neurobiol. 2009. PMID: 19552996 Review.
-
Organotypic cultures as tools for optimizing central nervous system cell therapies.Exp Neurol. 2013 Oct;248:429-40. doi: 10.1016/j.expneurol.2013.07.012. Epub 2013 Jul 27. Exp Neurol. 2013. PMID: 23899655 Review.
References
-
- Gähwiler BH. Organotypic monolayer cultures of nervous tissue. J Neurosci Methods. 1981;4:329‐342. - PubMed
-
- Gähwiler BH. Morphological differentiation of nerve cells in thin organotypic cultures derived from rat hippocampus and cerebellum. Proc R Soc Lond B Biol Sci. 1981b;211:287‐290. - PubMed
-
- Gähwiler BH. Organotypic cultures of neural tissue. Trends Neurosci. 1988;11:484‐489. - PubMed
-
- Stoppini L, Buchs PA, Muller D. A simple method for organotypic cultures of nervous tissue. J Neurosci Methods. 1991;37:173‐182. - PubMed
Publication types
MeSH terms
Substances
LinkOut - more resources
Full Text Sources
Research Materials