The intralumenal fragment pathway mediates ESCRT-independent surface transporter down-regulation
- PMID: 30560896
- PMCID: PMC6299085
- DOI: 10.1038/s41467-018-07734-5
The intralumenal fragment pathway mediates ESCRT-independent surface transporter down-regulation
Abstract
Surface receptor and transporter protein down-regulation is assumed to be exclusively mediated by the canonical multivesicular body (MVB) pathway and ESCRTs (Endosomal Sorting Complexes Required for Transport). However, few surface proteins are known to require ESCRTs for down-regulation, and reports of ESCRT-independent degradation are emerging, suggesting that alternative pathways exist. Here, using Saccharomyces cerevisiae as a model, we show that the hexose transporter Hxt3 does not require ESCRTs for down-regulation conferring resistance to 2-deoxyglucose. This is consistent with GFP-tagged Hxt3 bypassing ESCRT-mediated entry into intralumenal vesicles at endosomes. Instead, Hxt3-GFP accumulates on vacuolar lysosome membranes and is sorted into an area that, upon fusion, is internalized as an intralumenal fragment (ILF) and degraded. Moreover, heat stress or cycloheximide trigger degradation of Hxt3-GFP and other surface transporter proteins (Itr1, Aqr1) by this ESCRT-independent process. How this ILF pathway compares to the MVB pathway and potentially contributes to physiology is discussed.
Conflict of interest statement
The authors declare no competing interests.
Figures
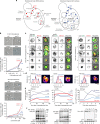
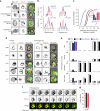
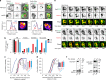
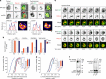
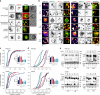
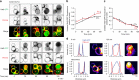
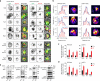
Similar articles
-
ESCRT, not intralumenal fragments, sorts ubiquitinated vacuole membrane proteins for degradation.J Cell Biol. 2021 Aug 2;220(8):e202012104. doi: 10.1083/jcb.202012104. Epub 2021 May 28. J Cell Biol. 2021. PMID: 34047770 Free PMC article.
-
Vps27 recruits ESCRT machinery to endosomes during MVB sorting.J Cell Biol. 2003 Aug 4;162(3):413-23. doi: 10.1083/jcb.200302136. J Cell Biol. 2003. PMID: 12900393 Free PMC article.
-
A two-tiered system for selective receptor and transporter protein degradation.PLoS Genet. 2022 Oct 10;18(10):e1010446. doi: 10.1371/journal.pgen.1010446. eCollection 2022 Oct. PLoS Genet. 2022. PMID: 36215320 Free PMC article.
-
Developmental and cellular functions of the ESCRT machinery in pluricellular organisms.Biol Cell. 2010 Jan 13;102(3):191-202. doi: 10.1042/BC20090145. Biol Cell. 2010. PMID: 20059450 Review.
-
ESCRT-III on endosomes: new functions, new activation pathway.Biochem J. 2016 Jan 15;473(2):e5-8. doi: 10.1042/BJ20151115. Biochem J. 2016. PMID: 26733719 Review.
Cited by
-
Lysosome Depletion-Triggered Autophagy Impairment in Progressive Kidney Injury.Kidney Dis (Basel). 2021 Jul;7(4):254-267. doi: 10.1159/000515035. Epub 2021 May 25. Kidney Dis (Basel). 2021. PMID: 34395541 Free PMC article. Review.
-
Exosomal cargoes in OSCC: current findings and potential functions.PeerJ. 2020 Nov 3;8:e10062. doi: 10.7717/peerj.10062. eCollection 2020. PeerJ. 2020. PMID: 33194377 Free PMC article.
-
Engineering stem cells to produce exosomes with enhanced bone regeneration effects: an alternative strategy for gene therapy.J Nanobiotechnology. 2022 Mar 15;20(1):135. doi: 10.1186/s12951-022-01347-3. J Nanobiotechnology. 2022. PMID: 35292020 Free PMC article.
-
Focus on the morphogenesis, fate and the role in tumor progression of multivesicular bodies.Cell Commun Signal. 2020 Aug 8;18(1):122. doi: 10.1186/s12964-020-00619-5. Cell Commun Signal. 2020. PMID: 32771015 Free PMC article. Review.
-
LAMTOR1 decreased exosomal PD-L1 to enhance immunotherapy efficacy in non-small cell lung cancer.Mol Cancer. 2024 Sep 2;23(1):184. doi: 10.1186/s12943-024-02099-4. Mol Cancer. 2024. PMID: 39223601 Free PMC article.
References
Publication types
MeSH terms
Substances
LinkOut - more resources
Full Text Sources
Molecular Biology Databases