A Mechanically Weak Extracellular Membrane-Adjacent Domain Induces Dimerization of Protocadherin-15
- PMID: 30527337
- PMCID: PMC6302040
- DOI: 10.1016/j.bpj.2018.11.010
A Mechanically Weak Extracellular Membrane-Adjacent Domain Induces Dimerization of Protocadherin-15
Abstract
The cadherin superfamily of proteins is defined by the presence of extracellular cadherin (EC) "repeats" that engage in protein-protein interactions to mediate cell-cell adhesion, cell signaling, and mechanotransduction. The extracellular domains of nonclassical cadherins often have a large number of EC repeats along with other subdomains of various folds. Protocadherin-15 (PCDH15), a protein component of the inner-ear tip link filament essential for mechanotransduction, has 11 EC repeats and a membrane adjacent domain (MAD12) of atypical fold. Here we report the crystal structure of a pig PCDH15 fragment including EC10, EC11, and MAD12 in a parallel dimeric arrangement. MAD12 has a unique molecular architecture and folds as a ferredoxin-like domain similar to that found in the nucleoporin protein Nup54. Analytical ultracentrifugation experiments along with size-exclusion chromatography coupled to multiangle laser light scattering and small-angle x-ray scattering corroborate the crystallographic dimer and show that MAD12 induces parallel dimerization of PCDH15 near its membrane insertion point. In addition, steered molecular dynamics simulations suggest that MAD12 is mechanically weak and may unfold before tip-link rupture. Sequence analyses and structural modeling predict the existence of similar domains in cadherin-23, protocadherin-24, and the "giant" FAT and CELSR cadherins, indicating that some of them may also exhibit MAD-induced parallel dimerization.
Copyright © 2018 Biophysical Society. Published by Elsevier Inc. All rights reserved.
Figures
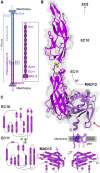
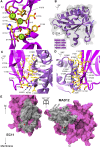
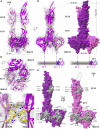
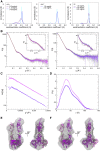
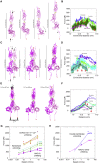
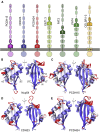
Similar articles
-
An elastic element in the protocadherin-15 tip link of the inner ear.Nat Commun. 2016 Nov 18;7:13458. doi: 10.1038/ncomms13458. Nat Commun. 2016. PMID: 27857071 Free PMC article.
-
Tuning Inner-Ear Tip-Link Affinity Through Alternatively Spliced Variants of Protocadherin-15.Biochemistry. 2018 Mar 20;57(11):1702-1710. doi: 10.1021/acs.biochem.7b01075. Epub 2018 Mar 6. Biochemistry. 2018. PMID: 29443515 Free PMC article.
-
Structure of a force-conveying cadherin bond essential for inner-ear mechanotransduction.Nature. 2012 Dec 6;492(7427):128-32. doi: 10.1038/nature11590. Epub 2012 Nov 7. Nature. 2012. PMID: 23135401 Free PMC article.
-
Cadherins and mechanotransduction by hair cells.Curr Opin Cell Biol. 2008 Oct;20(5):557-66. doi: 10.1016/j.ceb.2008.06.004. Epub 2008 Jul 30. Curr Opin Cell Biol. 2008. PMID: 18619539 Free PMC article. Review.
-
The tip-link molecular complex of the auditory mechano-electrical transduction machinery.Hear Res. 2015 Dec;330(Pt A):10-7. doi: 10.1016/j.heares.2015.05.005. Epub 2015 Jun 3. Hear Res. 2015. PMID: 26049141 Review.
Cited by
-
Collective mechanical responses of cadherin-based adhesive junctions as predicted by simulations.Biophys J. 2022 Mar 15;121(6):991-1012. doi: 10.1016/j.bpj.2022.02.008. Epub 2022 Feb 10. Biophys J. 2022. PMID: 35150618 Free PMC article.
-
A toolkit for studying cell surface shedding of diverse transmembrane receptors.Elife. 2019 Jun 7;8:e46983. doi: 10.7554/eLife.46983. Elife. 2019. PMID: 31172946 Free PMC article.
-
PKHD1L1 is required for stereocilia bundle maintenance, durable hearing function and resilience to noise exposure.bioRxiv [Preprint]. 2024 Mar 27:2024.02.29.582786. doi: 10.1101/2024.02.29.582786. bioRxiv. 2024. Update in: Commun Biol. 2024 Nov 1;7(1):1423. doi: 10.1038/s42003-024-07121-5 PMID: 38496629 Free PMC article. Updated. Preprint.
-
Single-molecule force spectroscopy reveals the dynamic strength of the hair-cell tip-link connection.Nat Commun. 2021 Feb 8;12(1):849. doi: 10.1038/s41467-021-21033-6. Nat Commun. 2021. PMID: 33558532 Free PMC article.
-
Mini-PCDH15 gene therapy rescues hearing in a mouse model of Usher syndrome type 1F.Nat Commun. 2023 Apr 26;14(1):2400. doi: 10.1038/s41467-023-38038-y. Nat Commun. 2023. PMID: 37100771 Free PMC article.
References
Publication types
MeSH terms
Substances
Grants and funding
LinkOut - more resources
Full Text Sources