A Pipeline for Volume Electron Microscopy of the Caenorhabditis elegans Nervous System
- PMID: 30524248
- PMCID: PMC6262311
- DOI: 10.3389/fncir.2018.00094
A Pipeline for Volume Electron Microscopy of the Caenorhabditis elegans Nervous System
Erratum in
-
Corrigendum: A Pipeline for Volume Electron Microscopy of the Caenorhabditis elegans Nervous System.Front Neural Circuits. 2019 Mar 20;13:16. doi: 10.3389/fncir.2019.00016. eCollection 2019. Front Neural Circuits. 2019. PMID: 30949033 Free PMC article.
Abstract
The "connectome," a comprehensive wiring diagram of synaptic connectivity, is achieved through volume electron microscopy (vEM) analysis of an entire nervous system and all associated non-neuronal tissues. White et al. (1986) pioneered the fully manual reconstruction of a connectome using Caenorhabditis elegans. Recent advances in vEM allow mapping new C. elegans connectomes with increased throughput, and reduced subjectivity. Current vEM studies aim to not only fill the remaining gaps in the original connectome, but also address fundamental questions including how the connectome changes during development, the nature of individuality, sexual dimorphism, and how genetic and environmental factors regulate connectivity. Here we describe our current vEM pipeline and projected improvements for the study of the C. elegans nervous system and beyond.
Keywords: C. elegans; connectome; high-pressure freezing; nervous system; volume electron microscopy.
Figures
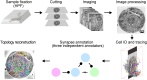
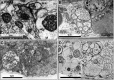
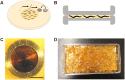
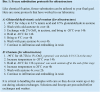
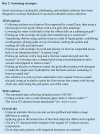
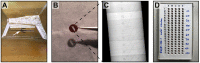
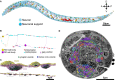
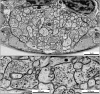
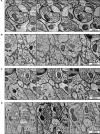
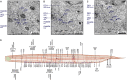
Similar articles
-
The Worm Connectome: Back to the Future.Trends Neurosci. 2018 Nov;41(11):763-765. doi: 10.1016/j.tins.2018.09.002. Trends Neurosci. 2018. PMID: 30366562
-
Whole-animal connectomes of both Caenorhabditis elegans sexes.Nature. 2019 Jul;571(7763):63-71. doi: 10.1038/s41586-019-1352-7. Epub 2019 Jul 3. Nature. 2019. PMID: 31270481 Free PMC article.
-
The connectome of a decision-making neural network.Science. 2012 Jul 27;337(6093):437-44. doi: 10.1126/science.1221762. Science. 2012. PMID: 22837521
-
Caenorhabditis elegans and the network control framework-FAQs.Philos Trans R Soc Lond B Biol Sci. 2018 Sep 10;373(1758):20170372. doi: 10.1098/rstb.2017.0372. Philos Trans R Soc Lond B Biol Sci. 2018. PMID: 30201837 Free PMC article. Review.
-
Semi-automated reconstruction of neural circuits using electron microscopy.Curr Opin Neurobiol. 2010 Oct;20(5):667-75. doi: 10.1016/j.conb.2010.08.002. Epub 2010 Sep 15. Curr Opin Neurobiol. 2010. PMID: 20833533 Review.
Cited by
-
C. elegans neurons have functional dendritic spines.Elife. 2019 Oct 4;8:e47918. doi: 10.7554/eLife.47918. Elife. 2019. PMID: 31584430 Free PMC article.
-
Neural Tissue Homeostasis and Repair Is Regulated via CS and DS Proteoglycan Motifs.Front Cell Dev Biol. 2021 Aug 2;9:696640. doi: 10.3389/fcell.2021.696640. eCollection 2021. Front Cell Dev Biol. 2021. PMID: 34409033 Free PMC article. Review.
-
Disruption of mitochondrial dynamics affects behaviour and lifespan in Caenorhabditis elegans.Cell Mol Life Sci. 2019 May;76(10):1967-1985. doi: 10.1007/s00018-019-03024-5. Epub 2019 Mar 6. Cell Mol Life Sci. 2019. PMID: 30840087 Free PMC article.
-
CaMKII mediates sexually dimorphic synaptic transmission at neuromuscular junctions in C. elegans.J Cell Biol. 2023 Nov 6;222(11):e202301117. doi: 10.1083/jcb.202301117. Epub 2023 Aug 25. J Cell Biol. 2023. PMID: 37624117 Free PMC article.
-
Cryo-fluorescence microscopy of high-pressure frozen C. elegans enables correlative FIB-SEM imaging of targeted embryonic stages in the intact worm.Methods Cell Biol. 2021;162:223-252. doi: 10.1016/bs.mcb.2020.09.009. Epub 2020 Nov 4. Methods Cell Biol. 2021. PMID: 33707014 Free PMC article.
References
-
- Altun Z. F., Herndon L. A., Wolkow C. A., Crocker C., Lints R., Hall D. H. (2002–2018). WormAtlas. Available at: http://www.wormatlas.org.