Predicting Intraserotypic Recombination in Enterovirus 71
- PMID: 30487277
- PMCID: PMC6364027
- DOI: 10.1128/JVI.02057-18
Predicting Intraserotypic Recombination in Enterovirus 71
Abstract
Enteroviruses are well known for their ability to cause neurological damage and paralysis. The model enterovirus is poliovirus (PV), the causative agent of poliomyelitis, a condition characterized by acute flaccid paralysis. A related virus, enterovirus 71 (EV-A71), causes similar clinical outcomes in recurrent outbreaks throughout Asia. Retrospective phylogenetic analysis has shown that recombination between circulating strains of EV-A71 produces the outbreak-associated strains which exhibit increased virulence and/or transmissibility. While studies on the mechanism(s) of recombination in PV are ongoing in several laboratories, little is known about factors that influence recombination in EV-A71. We have developed a cell-based assay to study recombination of EV-A71 based upon previously reported assays for poliovirus recombination. Our results show that (i) EV-A71 strain type and RNA sequence diversity impacts recombination frequency in a predictable manner that mimics the observations found in nature; (ii) recombination is primarily a replicative process mediated by the RNA-dependent RNA polymerase; (iii) a mutation shown to reduce recombination in PV (L420A) similarly reduces EV-A71 recombination, suggesting conservation in mechanism(s); and (iv) sequencing of intraserotypic recombinant genomes indicates that template switching occurs by a mechanism that may require some sequence homology at the recombination junction and that the triggers for template switching may be sequence independent. The development of this recombination assay will permit further investigation on the interplay between replication, recombination and disease.IMPORTANCE Recombination is a mechanism that contributes to genetic diversity. We describe the first assay to study EV-A71 recombination. Results from this assay mimic what is observed in nature and can be used by others to predict future recombination events within the enterovirus species A group. In addition, our results highlight the central role played by the viral RNA-dependent RNA polymerase (RdRp) in the recombination process. Further, our results show that changes to a conserved residue in the RdRp from different species groups have a similar impact on viable recombinant virus yields, which is indicative of conservation in mechanism.
Keywords: EV-A71; conservation; predictive; replicative recombination.
Copyright © 2019 American Society for Microbiology.
Figures
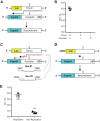
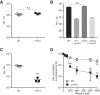
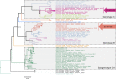
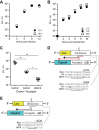
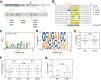
Similar articles
-
High Permissiveness for Genetic Exchanges between Enteroviruses of Species A, including Enterovirus 71, Favors Evolution through Intertypic Recombination in Madagascar.J Virol. 2019 Mar 5;93(6):e01667-18. doi: 10.1128/JVI.01667-18. Print 2019 Mar 15. J Virol. 2019. PMID: 30602612 Free PMC article.
-
Virulence of Enterovirus A71 Fluctuates Depending on the Phylogenetic Clade Formed in the Epidemic Year and Epidemic Region.J Virol. 2021 Nov 9;95(23):e0151521. doi: 10.1128/JVI.01515-21. Epub 2021 Sep 15. J Virol. 2021. PMID: 34523967 Free PMC article.
-
Next generation sequencing of human enterovirus strains from an outbreak of enterovirus A71 shows applicability to outbreak investigations.J Clin Virol. 2020 Jan;122:104216. doi: 10.1016/j.jcv.2019.104216. Epub 2019 Nov 17. J Clin Virol. 2020. PMID: 31790967 Free PMC article.
-
Changes in the EV-A71 Genome through Recombination and Spontaneous Mutations: Impact on Virulence.Viruses. 2018 Jun 12;10(6):320. doi: 10.3390/v10060320. Viruses. 2018. PMID: 29895721 Free PMC article. Review.
-
Viral determinants that drive Enterovirus-A71 fitness and virulence.Emerg Microbes Infect. 2021 Dec;10(1):713-724. doi: 10.1080/22221751.2021.1906754. Emerg Microbes Infect. 2021. PMID: 33745413 Free PMC article. Review.
Cited by
-
Substantial viral diversity in bats and rodents from East Africa: insights into evolution, recombination, and cocirculation.Microbiome. 2024 Apr 10;12(1):72. doi: 10.1186/s40168-024-01782-4. Microbiome. 2024. PMID: 38600530 Free PMC article.
-
Enterovirus A71: virulence, antigenicity, and genetic evolution over the years.J Biomed Sci. 2019 Oct 21;26(1):81. doi: 10.1186/s12929-019-0574-1. J Biomed Sci. 2019. PMID: 31630680 Free PMC article. Review.
-
The coronavirus proofreading exoribonuclease mediates extensive viral recombination.PLoS Pathog. 2021 Jan 19;17(1):e1009226. doi: 10.1371/journal.ppat.1009226. eCollection 2021 Jan. PLoS Pathog. 2021. PMID: 33465137 Free PMC article.
-
RNA-Dependent RNA Polymerase Speed and Fidelity are not the Only Determinants of the Mechanism or Efficiency of Recombination.Genes (Basel). 2019 Nov 25;10(12):968. doi: 10.3390/genes10120968. Genes (Basel). 2019. PMID: 31775299 Free PMC article.
-
Foot-and-Mouth Disease Virus Interserotypic Recombination in Superinfected Carrier Cattle.Pathogens. 2022 Jun 3;11(6):644. doi: 10.3390/pathogens11060644. Pathogens. 2022. PMID: 35745498 Free PMC article.
References
-
- Adams MJ, Lefkowitz EJ, King AMQ, Bamford DH, Breitbart M, Davison AJ, Ghabrial SA, Gorbalenya AE, Knowles NJ, Krell P, Lavigne R, Prangishvili D, Sanfacon H, Siddell SG, Simmonds P, Carstens EB. 2015. Ratification vote on taxonomic proposals to the International Committee on Taxonomy of Viruses. Arch Virol 160:1837–1850. doi:10.1007/s00705-015-2425-z. - DOI - PubMed
-
- Domingo E, Baranowski E, Escarmis C, Sobrino F, Holland JJ. 2002. Error frequencies of picornavirus RNA polymerases: evolutionary implications for virus populations, p 285–298. In Semler B, Wimmer E (ed), Molecular Biology of Picornavirus. ASM Press, Washington, DC. doi:10.1128/9781555817916.ch23. - DOI
Publication types
MeSH terms
Grants and funding
LinkOut - more resources
Full Text Sources
Research Materials