PARP family enzymes: regulation and catalysis of the poly(ADP-ribose) posttranslational modification
- PMID: 30481609
- PMCID: PMC6687463
- DOI: 10.1016/j.sbi.2018.11.002
PARP family enzymes: regulation and catalysis of the poly(ADP-ribose) posttranslational modification
Abstract
Poly(ADP-ribose) is a posttranslational modification and signaling molecule that regulates many aspects of human cell biology, and it is synthesized by enzymes known as poly(ADP-ribose) polymerases, or PARPs. A diverse collection of domain structures dictates the different cellular roles of PARP enzymes and regulates the production of poly(ADP-ribose). Here we primarily review recent structural insights into the regulation and catalysis of two family members: PARP-1 and Tankyrase. PARP-1 has multiple roles in the cellular response to DNA damage and the regulation of gene transcription, and Tankyrase regulates a diverse set of target proteins involved in cellular processes such as mitosis, genome integrity, and cell signaling. Both enzymes offer interesting modes of regulating the production and the target site selectivity of the poly(ADP-ribose) modification.
Copyright © 2018 Elsevier Ltd. All rights reserved.
Conflict of interest statement
Conflict of interest
None declared.
Figures
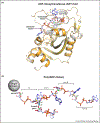
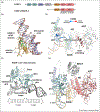
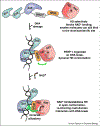
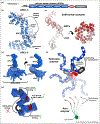
Similar articles
-
Poly(ADP-ribose) polymerase enzymes and the maintenance of genome integrity.Cell Mol Life Sci. 2020 Jan;77(1):19-33. doi: 10.1007/s00018-019-03366-0. Epub 2019 Nov 21. Cell Mol Life Sci. 2020. PMID: 31754726 Free PMC article. Review.
-
Regulation of PARP1/2 and the tankyrases: emerging parallels.Biochem J. 2024 Sep 4;481(17):1097-1123. doi: 10.1042/BCJ20230230. Biochem J. 2024. PMID: 39178157 Review.
-
Functional characterization of the poly(ADP-ribose) polymerase activity of tankyrase 1, a potential regulator of telomere length.J Mol Biol. 2002 Oct 18;323(2):217-24. doi: 10.1016/s0022-2836(02)00946-4. J Mol Biol. 2002. PMID: 12381316
-
PARP Power: A Structural Perspective on PARP1, PARP2, and PARP3 in DNA Damage Repair and Nucleosome Remodelling.Int J Mol Sci. 2021 May 12;22(10):5112. doi: 10.3390/ijms22105112. Int J Mol Sci. 2021. PMID: 34066057 Free PMC article. Review.
-
Poly (ADP-Ribose) Polymerases (PARPs) and PARP Inhibitor-Targeted Therapeutics.Anticancer Agents Med Chem. 2019;19(2):206-212. doi: 10.2174/1871520618666181109164645. Anticancer Agents Med Chem. 2019. PMID: 30417796 Review.
Cited by
-
SARS-CoV-2 and the DNA damage response.J Gen Virol. 2023 Nov;104(11):001918. doi: 10.1099/jgv.0.001918. J Gen Virol. 2023. PMID: 37948194 Free PMC article. Review.
-
Role of Poly [ADP-ribose] Polymerase 1 in Activating the Kirsten ras (KRAS) Gene in Response to Oxidative Stress.Int J Mol Sci. 2020 Aug 28;21(17):6237. doi: 10.3390/ijms21176237. Int J Mol Sci. 2020. PMID: 32872305 Free PMC article.
-
PARP inhibitors: shifting the paradigm in the treatment of pancreatic cancer.Med Oncol. 2021 Apr 23;38(6):61. doi: 10.1007/s12032-021-01507-9. Med Oncol. 2021. PMID: 33891252 Review.
-
Targets of Immune Escape Mechanisms in Cancer: Basis for Development and Evolution of Cancer Immune Checkpoint Inhibitors.Biology (Basel). 2023 Jan 30;12(2):218. doi: 10.3390/biology12020218. Biology (Basel). 2023. PMID: 36829496 Free PMC article. Review.
-
A novel conserved family of Macro-like domains-putative new players in ADP-ribosylation signaling.PeerJ. 2019 May 1;7:e6863. doi: 10.7717/peerj.6863. eCollection 2019. PeerJ. 2019. PMID: 31106069 Free PMC article.
References
-
- Hottiger MO, Hassa PO, Lüscher B, Schüler H, Koch-Nolte F: Toward a unified nomenclature for mammalian ADP-ribosyltransferases. Trends Biochem Sci 2010, 35:208–219. - PubMed
Publication types
MeSH terms
Substances
Grants and funding
LinkOut - more resources
Full Text Sources
Miscellaneous