In vitro reconstitution of DNA replication initiated by genetic recombination: a T4 bacteriophage model for a type of DNA synthesis important for all cells
- PMID: 30403545
- PMCID: PMC6337909
- DOI: 10.1091/mbc.E18-06-0386
In vitro reconstitution of DNA replication initiated by genetic recombination: a T4 bacteriophage model for a type of DNA synthesis important for all cells
Abstract
Using a mixture of 10 purified DNA replication and DNA recombination proteins encoded by the bacteriophage T4 genome, plus two homologous DNA molecules, we have reconstituted the genetic recombination-initiated pathway that initiates DNA replication forks at late times of T4 bacteriophage infection. Inside the cell, this recombination-dependent replication (RDR) is needed to produce the long concatemeric T4 DNA molecules that serve as substrates for packaging the shorter, genome-sized viral DNA into phage heads. The five T4 proteins that catalyze DNA synthesis on the leading strand, plus the proteins required for lagging-strand DNA synthesis, are essential for the reaction, as are a special mediator protein (gp59) and a Rad51/RecA analogue (the T4 UvsX strand-exchange protein). Related forms of RDR are widespread in living organisms-for example, they play critical roles in the homologous recombination events that can restore broken ends of the DNA double helix, restart broken DNA replication forks, and cross over chromatids during meiosis in eukaryotes. Those processes are considerably more complex, and the results presented here should be informative for dissecting their detailed mechanisms.
Figures
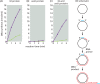
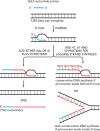
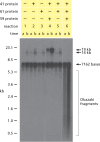
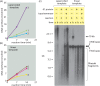
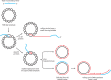
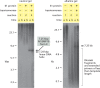
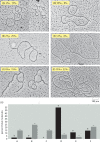
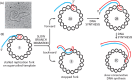
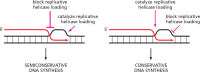
Similar articles
-
Mediator proteins orchestrate enzyme-ssDNA assembly during T4 recombination-dependent DNA replication and repair.Proc Natl Acad Sci U S A. 2001 Jul 17;98(15):8298-305. doi: 10.1073/pnas.131007498. Proc Natl Acad Sci U S A. 2001. PMID: 11459967 Free PMC article. Review.
-
The tight linkage between DNA replication and double-strand break repair in bacteriophage T4.Proc Natl Acad Sci U S A. 2001 Jul 17;98(15):8290-7. doi: 10.1073/pnas.131007598. Proc Natl Acad Sci U S A. 2001. PMID: 11459966 Free PMC article. Review.
-
Control of helicase loading in the coupled DNA replication and recombination systems of bacteriophage T4.J Biol Chem. 2014 Jan 31;289(5):3040-54. doi: 10.1074/jbc.M113.505842. Epub 2013 Dec 14. J Biol Chem. 2014. PMID: 24338568 Free PMC article.
-
Recombination-dependent DNA replication stimulated by double-strand breaks in bacteriophage T4.J Bacteriol. 1995 Dec;177(23):6844-53. doi: 10.1128/jb.177.23.6844-6853.1995. J Bacteriol. 1995. PMID: 7592477 Free PMC article.
-
Assembly and dynamics of Gp59-Gp32-single-stranded DNA (ssDNA), a DNA helicase loading complex required for recombination-dependent replication in bacteriophage T4.J Biol Chem. 2012 Jun 1;287(23):19070-81. doi: 10.1074/jbc.M112.343830. Epub 2012 Apr 12. J Biol Chem. 2012. PMID: 22500043 Free PMC article.
Cited by
-
Studies of Local DNA Backbone Conformation and Conformational Disorder Using Site-Specific Exciton-Coupled Dimer Probe Spectroscopy.Annu Rev Phys Chem. 2023 Apr 24;74:245-265. doi: 10.1146/annurev-physchem-090419-041204. Epub 2023 Jan 25. Annu Rev Phys Chem. 2023. PMID: 36696590 Free PMC article. Review.
-
Jumbo Phages: A Comparative Genomic Overview of Core Functions and Adaptions for Biological Conflicts.Viruses. 2021 Jan 5;13(1):63. doi: 10.3390/v13010063. Viruses. 2021. PMID: 33466489 Free PMC article.
-
Spectroscopic approaches for studies of site-specific DNA base and backbone 'breathing' using exciton-coupled dimer-labeled DNA.ArXiv [Preprint]. 2024 Mar 27:arXiv:2403.16251v2. ArXiv. 2024. PMID: 38584614 Free PMC article. Preprint.
References
-
- Alberts BM. (1984). The DNA enzymology of protein machines. Cold Spring Harb Symp Quant Biol , 1–12. - PubMed
-
- Alberts BM. (1998). The cell as a collection of protein machines: preparing the next generation of molecular biologists. Cell , 291–294. - PubMed
-
- Alberts BM, Barry J, Bedinger P, Formosa T, Jongeneel CV, Kreuzer KN. (1983). Studies on DNA replication in the bacteriophage T4 in vitro system. Cold Spring Harb Symp Quant Biol , 655–668. - PubMed
-
- Alberts BM, Frey L. (1970). T4 bacteriophage gene 32: a structural protein in the replication and recombination of DNA. Nature , 1313–1318. - PubMed
-
- Ando RA, Morrical SW. (1998). Single-stranded DNA binding properties of the UvsX recombinase of bacteriophage T4: binding parameters and effects of nucleotides. J Mol Biol , 785–796. - PubMed
Publication types
MeSH terms
Substances
Grants and funding
LinkOut - more resources
Full Text Sources
Other Literature Sources
Research Materials