TRIAMF: A New Method for Delivery of Cas9 Ribonucleoprotein Complex to Human Hematopoietic Stem Cells
- PMID: 30389991
- PMCID: PMC6214993
- DOI: 10.1038/s41598-018-34601-6
TRIAMF: A New Method for Delivery of Cas9 Ribonucleoprotein Complex to Human Hematopoietic Stem Cells
Abstract
CRISPR/Cas9 mediated gene editing of patient-derived hematopoietic stem and progenitor cells (HSPCs) ex vivo followed by autologous transplantation of the edited HSPCs back to the patient can provide a potential cure for monogenic blood disorders such as β-hemoglobinopathies. One challenge for this strategy is efficient delivery of the ribonucleoprotein (RNP) complex, consisting of purified Cas9 protein and guide RNA, into HSPCs. Because β-hemoglobinopathies are most prevalent in developing countries, it is desirable to have a reliable, efficient, easy-to-use and cost effective delivery method. With this goal in mind, we developed TRansmembrane Internalization Assisted by Membrane Filtration (TRIAMF), a new method to quickly and effectively deliver RNPs into HSPCs by passing a RNP and cell mixture through a filter membrane. We achieved robust gene editing in HSPCs using TRIAMF and demonstrated that the multilineage colony forming capacities and the competence for engraftment in immunocompromised mice of HSPCs were preserved post TRIAMF treatment. TRIAMF is a custom designed system using inexpensive components and has the capacity to process HSPCs at clinical scale.
Conflict of interest statement
At the time the research was performed, the authors were employees of Novartis. Several of the authors are inventors on patent applications owned by Novartis AG covering aspects of the work described in the manuscript.
Figures
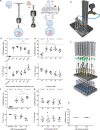
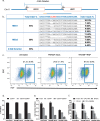
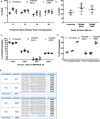
Similar articles
-
Optimization of CRISPR/Cas9 Delivery to Human Hematopoietic Stem and Progenitor Cells for Therapeutic Genomic Rearrangements.Mol Ther. 2019 Jan 2;27(1):137-150. doi: 10.1016/j.ymthe.2018.10.008. Epub 2018 Oct 17. Mol Ther. 2019. PMID: 30424953 Free PMC article.
-
Chemical Modification of Guide RNAs for Improved CRISPR Activity in CD34+ Human Hematopoietic Stem and Progenitor Cells.Methods Mol Biol. 2021;2162:37-48. doi: 10.1007/978-1-0716-0687-2_3. Methods Mol Biol. 2021. PMID: 32926376
-
Genome editing in human hematopoietic stem and progenitor cells via CRISPR-Cas9-mediated homology-independent targeted integration.Mol Ther. 2021 Apr 7;29(4):1611-1624. doi: 10.1016/j.ymthe.2020.12.010. Epub 2020 Dec 10. Mol Ther. 2021. PMID: 33309880 Free PMC article.
-
Recent advance on genome editing for therapy of β-hemoglobinopathies.Yi Chuan. 2018 Feb 20;40(2):95-103. doi: 10.16288/j.yczz.17-215. Yi Chuan. 2018. PMID: 29428902 Review.
-
Genome editing using CRISPR/Cas9 to treat hereditary hematological disorders.Gene Ther. 2022 May;29(5):207-216. doi: 10.1038/s41434-021-00247-9. Epub 2021 Mar 9. Gene Ther. 2022. PMID: 33750926 Review.
Cited by
-
CRISPR medicine for blood disorders: Progress and challenges in delivery.Front Genome Ed. 2023 Jan 6;4:1037290. doi: 10.3389/fgeed.2022.1037290. eCollection 2022. Front Genome Ed. 2023. PMID: 36687779 Free PMC article. Review.
-
Novelty in improvement of CAR T cell-based immunotherapy with the aid of CRISPR system.Hematol Transfus Cell Ther. 2024 Jan-Mar;46(1):58-66. doi: 10.1016/j.htct.2023.05.009. Epub 2023 Jul 12. Hematol Transfus Cell Ther. 2024. PMID: 37451978 Free PMC article. Review.
-
Visualizing the Nucleome Using the CRISPR-Cas9 System: From in vitro to in vivo.Biochemistry (Mosc). 2023 Jan;88(Suppl 1):S123-S149. doi: 10.1134/S0006297923140080. Biochemistry (Mosc). 2023. PMID: 37069118 Free PMC article. Review.
-
International Society for Cell & Gene Therapy Stem Cell Engineering Committee report on the current state of hematopoietic stem and progenitor cell-based genomic therapies and the challenges faced.Cytotherapy. 2024 Nov;26(11):1411-1420. doi: 10.1016/j.jcyt.2024.06.002. Epub 2024 Jun 6. Cytotherapy. 2024. PMID: 38970612
-
Protocol for assessment of the efficiency of CRISPR/Cas RNP delivery to different types of target cells.PLoS One. 2021 Nov 9;16(11):e0259812. doi: 10.1371/journal.pone.0259812. eCollection 2021. PLoS One. 2021. PMID: 34752487 Free PMC article.
References
MeSH terms
Substances
LinkOut - more resources
Full Text Sources
Other Literature Sources