Organizational principles of 3D genome architecture
- PMID: 30367165
- PMCID: PMC6312108
- DOI: 10.1038/s41576-018-0060-8
Organizational principles of 3D genome architecture
Abstract
Studies of 3D chromatin organization have suggested that chromosomes are hierarchically organized into large compartments composed of smaller domains called topologically associating domains (TADs). Recent evidence suggests that compartments are smaller than previously thought and that the transcriptional or chromatin state is responsible for interactions leading to the formation of small compartmental domains in all organisms. In vertebrates, CTCF forms loop domains, probably via an extrusion process involving cohesin. CTCF loops cooperate with compartmental domains to establish the 3D organization of the genome. The continuous extrusion of the chromatin fibre by cohesin may also be responsible for the establishment of enhancer-promoter interactions and stochastic aspects of the transcription process. These observations suggest that the 3D organization of the genome is an emergent property of chromatin and its components, and thus may not be only a determinant but also a consequence of its function.
Conflict of interest statement
Competing Interests
The authors declare no competing interests.
Figures
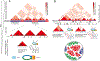
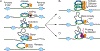
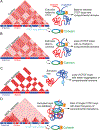
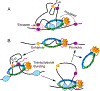
Similar articles
-
Chromatin organization by an interplay of loop extrusion and compartmental segregation.Proc Natl Acad Sci U S A. 2018 Jul 17;115(29):E6697-E6706. doi: 10.1073/pnas.1717730115. Epub 2018 Jul 2. Proc Natl Acad Sci U S A. 2018. PMID: 29967174 Free PMC article.
-
Recent evidence that TADs and chromatin loops are dynamic structures.Nucleus. 2018 Jan 1;9(1):20-32. doi: 10.1080/19491034.2017.1389365. Epub 2017 Dec 14. Nucleus. 2018. PMID: 29077530 Free PMC article. Review.
-
Impact of 3D genome organization, guided by cohesin and CTCF looping, on sex-biased chromatin interactions and gene expression in mouse liver.Epigenetics Chromatin. 2020 Jul 17;13(1):30. doi: 10.1186/s13072-020-00350-y. Epigenetics Chromatin. 2020. PMID: 32680543 Free PMC article.
-
Resolving the 3D Landscape of Transcription-Linked Mammalian Chromatin Folding.Mol Cell. 2020 May 7;78(3):539-553.e8. doi: 10.1016/j.molcel.2020.03.002. Epub 2020 Mar 25. Mol Cell. 2020. PMID: 32213323 Free PMC article.
-
Chromatin accessibility and the regulatory epigenome.Nat Rev Genet. 2019 Apr;20(4):207-220. doi: 10.1038/s41576-018-0089-8. Nat Rev Genet. 2019. PMID: 30675018 Review.
Cited by
-
SnapFISH-IMPUTE: an imputation method for multiplexed DNA FISH data.Commun Biol. 2024 Jul 9;7(1):834. doi: 10.1038/s42003-024-06428-7. Commun Biol. 2024. PMID: 38982263 Free PMC article.
-
Orchestrating chromosome conformation capture analysis with Bioconductor.Nat Commun. 2024 Feb 5;15(1):1072. doi: 10.1038/s41467-024-44761-x. Nat Commun. 2024. PMID: 38316789 Free PMC article.
-
Epigenetic regulation of intestinal stem cell differentiation.Am J Physiol Gastrointest Liver Physiol. 2020 Aug 1;319(2):G189-G196. doi: 10.1152/ajpgi.00084.2020. Epub 2020 Jul 6. Am J Physiol Gastrointest Liver Physiol. 2020. PMID: 32628072 Free PMC article. Review.
-
Chromatin-Spliceosome Mutations in Acute Myeloid Leukemia.Cancers (Basel). 2021 Mar 11;13(6):1232. doi: 10.3390/cancers13061232. Cancers (Basel). 2021. PMID: 33799787 Free PMC article. Review.
-
The macro and micro of chromosome conformation capture.Wiley Interdiscip Rev Dev Biol. 2021 Nov;10(6):e395. doi: 10.1002/wdev.395. Epub 2020 Sep 28. Wiley Interdiscip Rev Dev Biol. 2021. PMID: 32987449 Free PMC article. Review.
References
Publication types
MeSH terms
Substances
Grants and funding
LinkOut - more resources
Full Text Sources
Other Literature Sources