Developmental maturation of activity-induced K+ and pH transients and the associated extracellular space dynamics in the rat hippocampus
- PMID: 30357826
- PMCID: PMC6332761
- DOI: 10.1113/JP276768
Developmental maturation of activity-induced K+ and pH transients and the associated extracellular space dynamics in the rat hippocampus
Abstract
Key points: Neuronal activity induces fluctuation in extracellular space volume, [K+ ]o and pHo , the management of which influences neuronal function The neighbour astrocytes buffer the K+ and pH and swell during the process, causing shrinkage of the extracellular space In the present study, we report the developmental rise of the homeostatic control of the extracellular space dynamics, for which regulation becomes tighter with maturation and thus is proposed to ensure efficient synaptic transmission in the mature animals The extracellular space dynamics of volume, [K+ ]o and pHo evolve independently with developmental maturation and, although all of them are inextricably tied to neuronal activity, they do not couple directly.
Abstract: Neuronal activity in the mammalian central nervous system associates with transient extracellular space (ECS) dynamics involving elevated K+ and pH and shrinkage of the ECS. These ECS properties affect membrane potentials, neurotransmitter concentrations and protein function and are thus anticipated to be under tight regulatory control. It remains unresolved to what extent these ECS dynamics are developmentally regulated as synaptic precision arises and whether they are directly or indirectly coupled. To resolve the development of homeostatic control of [K+ ]o , pH, and ECS and their interaction, we utilized ion-sensitive microelectrodes in electrically stimulated rat hippocampal slices from rats of different developmental stages (postnatal days 3-28). With the employed stimulation paradigm, the stimulus-evoked peak [K+ ]o and pHo transients were stable across age groups, until normalized to neuronal activity (field potential amplitude), in which case the K+ and pH shifted significantly more in the younger animals. By contrast, ECS dynamics increased with age until normalized to the field potential, and thus correlated with neuronal activity. With age, the animals not only managed the peak [K+ ]o better, but also displayed swifter post-stimulus removal of [K+ ]o , in correlation with the increased expression of the α1-3 isoforms of the Na+ /K+ -ATPase, and a swifter return of ECS volume. The different ECS dynamics approached a near-identical temporal pattern in the more mature animals. In conclusion, although these phenomena are inextricably tied to neuronal activity, our data suggest that they do not couple directly.
Keywords: Extracellular space dynamics; K+ homeostasis; Na+/K+-ATPase; astrocytes; pH transients.
© 2018 The Authors. The Journal of Physiology © 2018 The Physiological Society.
Figures
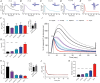
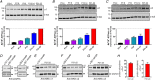
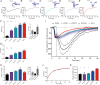
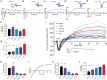
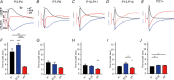
Similar articles
-
Activity-dependent astrocyte swelling is mediated by pH-regulating mechanisms.Glia. 2017 Oct;65(10):1668-1681. doi: 10.1002/glia.23187. Epub 2017 Jul 26. Glia. 2017. PMID: 28744903
-
Contributions of the Na⁺/K⁺-ATPase, NKCC1, and Kir4.1 to hippocampal K⁺ clearance and volume responses.Glia. 2014 Apr;62(4):608-22. doi: 10.1002/glia.22629. Epub 2014 Jan 30. Glia. 2014. PMID: 24482245 Free PMC article.
-
Glutamate transporter activity promotes enhanced Na+ /K+ -ATPase-mediated extracellular K+ management during neuronal activity.J Physiol. 2016 Nov 15;594(22):6627-6641. doi: 10.1113/JP272531. Epub 2016 Jun 29. J Physiol. 2016. PMID: 27231201 Free PMC article.
-
Glial diffusion barriers during aging and pathological states.Prog Brain Res. 2001;132:339-63. doi: 10.1016/S0079-6123(01)32087-3. Prog Brain Res. 2001. PMID: 11545002 Review.
-
Ionic changes and alterations in the size of the extracellular space during epileptic activity.Adv Neurol. 1986;44:619-39. Adv Neurol. 1986. PMID: 3518349 Review.
Cited by
-
Nanoscale and functional heterogeneity of the hippocampal extracellular space.Cell Rep. 2023 May 30;42(5):112478. doi: 10.1016/j.celrep.2023.112478. Epub 2023 May 5. Cell Rep. 2023. PMID: 37149864 Free PMC article.
-
ECS Dynamism and Its Influence on Neuronal Excitability and Seizures.Neurochem Res. 2019 May;44(5):1020-1036. doi: 10.1007/s11064-019-02773-w. Epub 2019 Mar 16. Neurochem Res. 2019. PMID: 30879174 Review.
-
On the origin of ultraslow spontaneous Na+ fluctuations in neurons of the neonatal forebrain.J Neurophysiol. 2021 Feb 1;125(2):408-425. doi: 10.1152/jn.00373.2020. Epub 2020 Nov 25. J Neurophysiol. 2021. PMID: 33236936 Free PMC article.
-
Molecular mechanisms of brain water transport.Nat Rev Neurosci. 2021 Jun;22(6):326-344. doi: 10.1038/s41583-021-00454-8. Epub 2021 Apr 12. Nat Rev Neurosci. 2021. PMID: 33846637 Review.
-
Rapid volume pulsation of the extracellular space coincides with epileptiform activity in mice and depends on the NBCe1 transporter.J Physiol. 2021 Jun;599(12):3195-3220. doi: 10.1113/JP281544. Epub 2021 May 29. J Physiol. 2021. PMID: 33942325 Free PMC article.
References
-
- Ballanyi K, Grafe P, Reddy MM & Ten Bruggencate G (1984). Different types of potassium transport linked to carbachol and γ‐aminobutyric acid actions in rat sympathetic neurons. Neuroscience 12, 917–927. - PubMed
-
- Bondareff W & Pysh JJ (1968). Distribution of the extracellular space during postnatal maturation of rat cerebral cortex. Anat Rec 160, 773–780. - PubMed
-
- Brown AM & Ransom BR (2015). Astrocyte glycogen as an emergency fuel under conditions of glucose deprivation or intense neural activity. Metab Brain Dis 30, 233–239. - PubMed
-
- Catania MV, Landwehrmeyer GB, Testa CM, Standaert DG, Penney JB & Young AB (1994). Metabotropic glutamate receptors are differentially regulated during development. Neuroscience 61, 481–495. - PubMed
Publication types
MeSH terms
Substances
LinkOut - more resources
Full Text Sources
Medical