Ultrastructural Analysis of Vesicular Transport in Electrotransfection
- PMID: 30334512
- PMCID: PMC6196718
- DOI: 10.1017/S143192761801509X
Ultrastructural Analysis of Vesicular Transport in Electrotransfection
Abstract
Emerging evidence from various studies indicates that plasmid DNA (pDNA) is internalized by cells through an endocytosis-like process when it is used for electrotransfection. To provide morphological evidence of the process, we investigated ultrastructures in cells that were associated with the electrotransfected pDNA, using immunoelectron microscopy. The results demonstrate that four endocytic pathways are involved in the uptake of the pDNA, including caveolae- and clathrin-mediated endocytosis, macropinocytosis, and the clathrin-independent carrier/glycosylphosphatidylinositol-anchored protein-enriched early endosomal compartment (CLIC/GEEC) pathway. Among them, macropinocytosis is the most common pathway utilized by cells having various pDNA uptake capacities, and the CLIC/GEEC pathway is observed primarily in human umbilical vein endothelial cells. Quantitatively, the endocytic pathways are more active in easy-to-transfect cells than in hard-to-transfect ones. Taken together, our data provide ultrastructural evidence showing that endocytosis plays an important role in cellular uptake and intracellular transport of electrotransfected pDNA.
Keywords: clathrin-mediated endocytosis; electroporation; electrotransfection; intracellular trafficking; macropinocytosis.
Figures
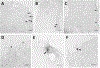
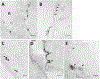
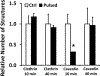
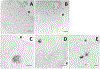
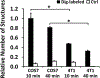
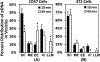
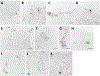
Similar articles
-
Involvement of a Rac1-Dependent Macropinocytosis Pathway in Plasmid DNA Delivery by Electrotransfection.Mol Ther. 2017 Mar 1;25(3):803-815. doi: 10.1016/j.ymthe.2016.12.009. Epub 2017 Jan 24. Mol Ther. 2017. PMID: 28129959 Free PMC article.
-
Endocytic crosstalk: cavins, caveolins, and caveolae regulate clathrin-independent endocytosis.PLoS Biol. 2014 Apr 8;12(4):e1001832. doi: 10.1371/journal.pbio.1001832. eCollection 2014 Apr. PLoS Biol. 2014. PMID: 24714042 Free PMC article.
-
Macropinocytosis of polyplexes and recycling of plasmid via the clathrin-dependent pathway impair the transfection efficiency of human hepatocarcinoma cells.Mol Ther. 2004 Aug;10(2):373-85. doi: 10.1016/j.ymthe.2004.05.023. Mol Ther. 2004. PMID: 15294184
-
Mechanisms of Carrier Formation during Clathrin-Independent Endocytosis.Trends Cell Biol. 2018 Mar;28(3):188-200. doi: 10.1016/j.tcb.2017.11.004. Epub 2017 Dec 11. Trends Cell Biol. 2018. PMID: 29241687 Review.
-
Unconventional endocytic mechanisms.Curr Opin Cell Biol. 2021 Aug;71:120-129. doi: 10.1016/j.ceb.2021.03.001. Epub 2021 Apr 13. Curr Opin Cell Biol. 2021. PMID: 33862329 Review.
Cited by
-
Role of Endocytosis Pathways in Electropermeablization of MCF7 Cells Using Low Voltage and High Frequency Electrochemotherapy.Cell J. 2021 Sep;23(4):445-450. doi: 10.22074/cellj.2021.7203. Epub 2021 Aug 29. Cell J. 2021. PMID: 34455720 Free PMC article.
-
Aluminum particles generated during millisecond electric pulse application enhance adenovirus-mediated gene transfer in L929 cells.Sci Rep. 2021 Sep 6;11(1):17725. doi: 10.1038/s41598-021-96781-y. Sci Rep. 2021. PMID: 34489497 Free PMC article.
-
A statistical framework for determination of minimal plasmid copy number required for transgene expression in mammalian cells.Bioelectrochemistry. 2021 Apr;138:107731. doi: 10.1016/j.bioelechem.2020.107731. Epub 2020 Dec 29. Bioelectrochemistry. 2021. PMID: 33434786 Free PMC article.
-
Pulsed electric field induces exocytosis and overexpression of MAGE antigens in melanoma.Sci Rep. 2024 May 31;14(1):12546. doi: 10.1038/s41598-024-63181-x. Sci Rep. 2024. PMID: 38822068 Free PMC article.
-
Pathophysiological Role of Caveolae in Hypertension.Front Med (Lausanne). 2019 Jul 10;6:153. doi: 10.3389/fmed.2019.00153. eCollection 2019. Front Med (Lausanne). 2019. PMID: 31355199 Free PMC article. Review.