Heat-stress triggers MAPK crosstalk to turn on the hyperosmotic response pathway
- PMID: 30310096
- PMCID: PMC6181916
- DOI: 10.1038/s41598-018-33203-6
Heat-stress triggers MAPK crosstalk to turn on the hyperosmotic response pathway
Abstract
Cells make decisions based on a combination of external and internal signals. In yeast, the high osmolarity response (HOG) is a mitogen-activated protein kinase (MAPK) pathway that responds to a variety of stimuli, and it is central to the general stress response. Here we studied the effect of heat-stress (HS) on HOG. Using live-cell reporters and genetics, we show that HS promotes Hog1 phosphorylation and Hog1-dependent gene expression, exclusively via the Sln1 phosphorelay branch, and that the strength of the activation is larger in yeast adapted to high external osmolarity. HS stimulation of HOG is indirect. First, we show that HS causes glycerol loss, necessary for HOG activation. Preventing glycerol efflux by deleting the glyceroporin FPS1 or its regulators RGC1 and ASK10/RGC2, or by increasing external glycerol, greatly reduced HOG activation. Second, we found that HOG stimulation by HS depended on the operation of a second MAPK pathway, the cell-wall integrity (CWI), a well-known mediator of HS, since inactivating Pkc1 or deleting the MAPK SLT2 greatly reduced HOG activation. Our data suggest that the main role of the CWI in this process is to stimulate glycerol loss. We found that in yeast expressing the constitutively open channel mutant (Fps1-Δ11), HOG activity was independent of Slt2. In summary, we suggest that HS causes a reduction in turgor due to the loss of glycerol and the accompanying water, and that this is what actually stimulates HOG. Thus, taken together, our findings highlight a central role for Fps1, and the metabolism of glycerol, in the communication between the yeast MAPK pathways, essential for survival and reproduction in changing environments.
Conflict of interest statement
The authors declare no competing interests.
Figures
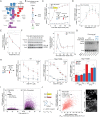
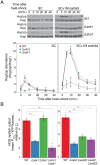
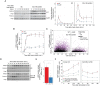
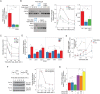
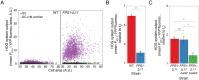
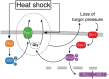
Similar articles
-
Putative Membrane Receptors Contribute to Activation and Efficient Signaling of Mitogen-Activated Protein Kinase Cascades during Adaptation of Aspergillus fumigatus to Different Stressors and Carbon Sources.mSphere. 2020 Sep 16;5(5):e00818-20. doi: 10.1128/mSphere.00818-20. mSphere. 2020. PMID: 32938702 Free PMC article.
-
Response to high osmotic conditions and elevated temperature in Saccharomyces cerevisiae is controlled by intracellular glycerol and involves coordinate activity of MAP kinase pathways.Microbiology (Reading). 2003 May;149(Pt 5):1193-1204. doi: 10.1099/mic.0.26110-0. Microbiology (Reading). 2003. PMID: 12724381
-
Identification of positive regulators of the yeast fps1 glycerol channel.PLoS Genet. 2009 Nov;5(11):e1000738. doi: 10.1371/journal.pgen.1000738. Epub 2009 Nov 26. PLoS Genet. 2009. PMID: 19956799 Free PMC article.
-
The high-osmolarity glycerol (HOG) and cell wall integrity (CWI) signalling pathways interplay: a yeast dialogue between MAPK routes.Yeast. 2010 Aug;27(8):495-502. doi: 10.1002/yea.1792. Yeast. 2010. PMID: 20641030 Review.
-
[Mechanism of HOG-MAPK pathway in regulating mycotoxins formation under environmental stresses].Sheng Wu Gong Cheng Xue Bao. 2022 Jul 25;38(7):2433-2446. doi: 10.13345/j.cjb.220060. Sheng Wu Gong Cheng Xue Bao. 2022. PMID: 35871615 Review. Chinese.
Cited by
-
Atg32-dependent mitophagy sustains spermidine and nitric oxide required for heat-stress tolerance in Saccharomycescerevisiae.J Cell Sci. 2021 Jun 1;134(11):jcs253781. doi: 10.1242/jcs.253781. Epub 2021 Jun 7. J Cell Sci. 2021. PMID: 34096604 Free PMC article.
-
Yeast lacking the sterol C-5 desaturase Erg3 are tolerant to the anti-inflammatory triterpenoid saponin escin.Sci Rep. 2023 Aug 21;13(1):13617. doi: 10.1038/s41598-023-40308-0. Sci Rep. 2023. PMID: 37604855 Free PMC article.
-
Proteomics Answers Which Yeast Genes Are Specific for Baking, Brewing, and Ethanol Production.Bioengineering (Basel). 2020 Nov 18;7(4):147. doi: 10.3390/bioengineering7040147. Bioengineering (Basel). 2020. PMID: 33217975 Free PMC article.
-
Engineering of Aspergillus niger for efficient production of D-xylitol from L-arabinose.Microb Cell Fact. 2024 Oct 5;23(1):262. doi: 10.1186/s12934-024-02526-7. Microb Cell Fact. 2024. PMID: 39367393 Free PMC article.
-
Effect of heat stress on mitogen-activated protein kinases in the hypothalamic-pituitary-gonadal axis of developing Wenchang chicks.Poult Sci. 2020 Jan;99(1):567-577. doi: 10.3382/ps/pez499. Epub 2019 Dec 30. Poult Sci. 2020. PMID: 32416843 Free PMC article.
References
Publication types
MeSH terms
Substances
LinkOut - more resources
Full Text Sources
Molecular Biology Databases
Research Materials