Insulin and 20-hydroxyecdysone oppose each other in the regulation of phosphoinositide-dependent kinase-1 expression during insect pupation
- PMID: 30305395
- PMCID: PMC6290142
- DOI: 10.1074/jbc.RA118.004891
Insulin and 20-hydroxyecdysone oppose each other in the regulation of phosphoinositide-dependent kinase-1 expression during insect pupation
Abstract
Insulin promotes larval growth of insects by stimulating the synthesis of the steroid hormone 20-hydroxyecdysone (20E), which induces pupation and apoptosis. However, the mechanism underlying the coordinate regulation of insect pupation and apoptosis by these two functionally opposing hormones is still unclear. Here, using the lepidopteran insect and serious agricultural pest Helicoverpa armigera (cotton bollworm) as a model, we report that phosphoinositide-dependent kinase-1 (PDK1) and forkhead box O (FoxO) play key roles in these processes. We found that the transcript levels of the PDK1 gene are increased during the larval feeding stages. Moreover, PDK1 expression was increased by insulin, but repressed by 20E. dsRNA-mediated PDK1 knockdown in the H. armigera larvae delayed pupation and resulted in small pupae and also decreased Akt/protein kinase B expression and increased FoxO expression. Furthermore, the PDK1 knockdown blocked midgut remodeling and decreased 20E levels in the larvae. Of note, injecting larvae with 20E overcame the effect of the PDK1 knockdown and restored midgut remodeling. FoxO overexpression in an H. armigera epidermal cell line (HaEpi) did not induce apoptosis, but promoted autophagy and repressed cell proliferation. These results reveal cross-talk between insulin and 20E and that both hormones oppose each other's activities in the regulation of insect pupation and apoptosis by controlling PDK1 expression and, in turn, FoxO expression. We conclude that sufficiently high 20E levels are a key factor for inducing apoptosis during insect pupation.
Keywords: 20-hydroxyecdysone; FoxO; PDK; apoptosis; autophagy; bollworm; cell proliferation; insect development; insulin; protein kinase; pupation; steroid hormone.
© 2018 Pan et al.
Conflict of interest statement
The authors declare that they have no conflicts of interest with the contents of this article
Figures
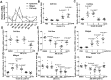
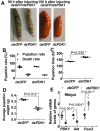
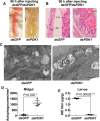
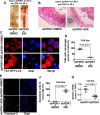
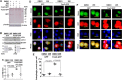
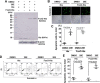
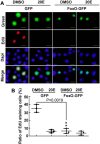
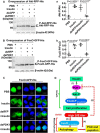
Similar articles
-
Research Progress on the Regulation of Autophagy and Apoptosis in Insects by Sterol Hormone 20-Hydroxyecdysone.Insects. 2023 Nov 12;14(11):871. doi: 10.3390/insects14110871. Insects. 2023. PMID: 37999070 Free PMC article. Review.
-
The steroid hormone 20-hydroxyecdysone induces phosphorylation and aggregation of stromal interacting molecule 1 for store-operated calcium entry.J Biol Chem. 2019 Oct 11;294(41):14922-14936. doi: 10.1074/jbc.RA119.008484. Epub 2019 Aug 14. J Biol Chem. 2019. PMID: 31413111 Free PMC article.
-
20-Hydroxyecdysone activates Forkhead box O to promote proteolysis during Helicoverpa armigera molting.Development. 2016 Mar 15;143(6):1005-15. doi: 10.1242/dev.128694. Epub 2016 Feb 18. Development. 2016. PMID: 26893349
-
The steroid hormone 20-hydroxyecdysone upregulates calcium release-activated calcium channel modulator 1 expression to induce apoptosis in the midgut of Helicoverpa armigera.Cell Calcium. 2017 Dec;68:24-33. doi: 10.1016/j.ceca.2017.10.004. Epub 2017 Oct 20. Cell Calcium. 2017. PMID: 29129205
-
Gregariousness in lepidopteran larvae.Insect Sci. 2024 Oct;31(5):1353-1364. doi: 10.1111/1744-7917.13312. Epub 2024 Jan 12. Insect Sci. 2024. PMID: 38214204 Review.
Cited by
-
Pupal Diapause Termination and Transcriptional Response of Antheraea pernyi (Lepidoptera: Saturniidae) Triggered by 20-Hydroxyecdysone.Front Physiol. 2022 May 26;13:888643. doi: 10.3389/fphys.2022.888643. eCollection 2022. Front Physiol. 2022. PMID: 35721532 Free PMC article.
-
Transcription and Post-translational Regulation of Autophagy in Insects.Front Physiol. 2022 Feb 25;13:825202. doi: 10.3389/fphys.2022.825202. eCollection 2022. Front Physiol. 2022. PMID: 35283796 Free PMC article.
-
Disruption of the Serine/Threonine Kinase Akt Gene Affects Ovarian Development and Fecundity in the Cigarette Beetle, Lasioderma serricorne.Front Physiol. 2021 Oct 7;12:765819. doi: 10.3389/fphys.2021.765819. eCollection 2021. Front Physiol. 2021. PMID: 34690822 Free PMC article.
-
Research Progress on the Regulation of Autophagy and Apoptosis in Insects by Sterol Hormone 20-Hydroxyecdysone.Insects. 2023 Nov 12;14(11):871. doi: 10.3390/insects14110871. Insects. 2023. PMID: 37999070 Free PMC article. Review.
-
Complex Evolution of Insect Insulin Receptors and Homologous Decoy Receptors, and Functional Significance of Their Multiplicity.Mol Biol Evol. 2020 Jun 1;37(6):1775-1789. doi: 10.1093/molbev/msaa048. Mol Biol Evol. 2020. PMID: 32101294 Free PMC article.
References
Publication types
MeSH terms
Substances
LinkOut - more resources
Full Text Sources
Medical
Miscellaneous