Role of KCa3.1 Channels in Modulating Ca2+ Oscillations during Glioblastoma Cell Migration and Invasion
- PMID: 30274242
- PMCID: PMC6213908
- DOI: 10.3390/ijms19102970
Role of KCa3.1 Channels in Modulating Ca2+ Oscillations during Glioblastoma Cell Migration and Invasion
Abstract
Cell migration and invasion in glioblastoma (GBM), the most lethal form of primary brain tumors, are critically dependent on Ca2+ signaling. Increases of [Ca2+]i in GBM cells often result from Ca2+ release from the endoplasmic reticulum (ER), promoted by a variety of agents present in the tumor microenvironment and able to activate the phospholipase C/inositol 1,4,5-trisphosphate PLC/IP₃ pathway. The Ca2+ signaling is further strengthened by the Ca2+ influx from the extracellular space through Ca2+ release-activated Ca2+ (CRAC) currents sustained by Orai/STIM channels, meant to replenish the partially depleted ER. Notably, the elevated cytosolic [Ca2+]i activates the intermediate conductance Ca2+-activated K (KCa3.1) channels highly expressed in the plasma membrane of GBM cells, and the resulting K⁺ efflux hyperpolarizes the cell membrane. This translates to an enhancement of Ca2+ entry through Orai/STIM channels as a result of the increased electromotive (driving) force on Ca2+ influx, ending with the establishment of a recurrent cycle reinforcing the Ca2+ signal. Ca2+ signaling in migrating GBM cells often emerges in the form of intracellular Ca2+ oscillations, instrumental to promote key processes in the migratory cycle. This has suggested that KCa3.1 channels may promote GBM cell migration by inducing or modulating the shape of Ca2+ oscillations. In accordance, we recently built a theoretical model of Ca2+ oscillations incorporating the KCa3.1 channel-dependent dynamics of the membrane potential, and found that the KCa3.1 channel activity could significantly affect the IP₃ driven Ca2+ oscillations. Here we review our new theoretical model of Ca2+ oscillations in GBM, upgraded in the light of better knowledge of the KCa3.1 channel kinetics and Ca2+ sensitivity, the dynamics of the Orai/STIM channel modulation, the migration and invasion mechanisms of GBM cells, and their regulation by Ca2+ signals.
Keywords: KCa3.1 channels; calcium oscillations; cell migration; glioblastoma; mathematical model.
Conflict of interest statement
The authors declare no conflict of interest.
Figures
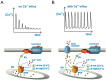
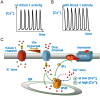
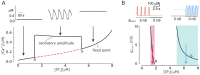
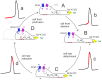
Similar articles
-
Store-Independent Orai Channels Regulated by STIM.In: Kozak JA, Putney JW Jr, editors. Calcium Entry Channels in Non-Excitable Cells. Boca Raton (FL): CRC Press/Taylor & Francis; 2018. Chapter 11. In: Kozak JA, Putney JW Jr, editors. Calcium Entry Channels in Non-Excitable Cells. Boca Raton (FL): CRC Press/Taylor & Francis; 2018. Chapter 11. PMID: 30299650 Free Books & Documents. Review.
-
KCa3.1 channels are involved in the infiltrative behavior of glioblastoma in vivo.Cell Death Dis. 2013 Aug 15;4(8):e773. doi: 10.1038/cddis.2013.279. Cell Death Dis. 2013. PMID: 23949222 Free PMC article.
-
Selective activation of KCa3.1 and CRAC channels by P2Y2 receptors promotes Ca(2+) signaling, store refilling and migration of rat microglial cells.PLoS One. 2013 Apr 19;8(4):e62345. doi: 10.1371/journal.pone.0062345. Print 2013. PLoS One. 2013. PMID: 23620825 Free PMC article.
-
KCa3.1 Channels and Glioblastoma: In Vitro Studies.Curr Neuropharmacol. 2018;16(5):627-635. doi: 10.2174/1570159X15666170808115821. Curr Neuropharmacol. 2018. PMID: 28786347 Free PMC article. Review.
-
Inhibition of KCa3.1 by depolarisation and 2-aminoethoxydiphenyl borate (2-APB) during Ca²⁺ release activated Ca²⁺ (CRAC) entry in human erythroleukemia (HEL) cells: Implications for the interpretation of 2-APB inhibition of CRAC entry.Cell Calcium. 2015 Feb;57(2):76-88. doi: 10.1016/j.ceca.2014.12.009. Epub 2014 Dec 23. Cell Calcium. 2015. PMID: 25601026
Cited by
-
KCNA1 promotes the growth and invasion of glioblastoma cells through ferroptosis inhibition via upregulating SLC7A11.Cancer Cell Int. 2024 Jan 3;24(1):7. doi: 10.1186/s12935-023-03199-9. Cancer Cell Int. 2024. PMID: 38172959 Free PMC article.
-
Tumor Cell Infiltration into the Brain in Glioblastoma: From Mechanisms to Clinical Perspectives.Cancers (Basel). 2022 Jan 17;14(2):443. doi: 10.3390/cancers14020443. Cancers (Basel). 2022. PMID: 35053605 Free PMC article. Review.
-
Ion Channels and Their Role in the Pathophysiology of Gliomas.Mol Cancer Ther. 2020 Oct;19(10):1959-1969. doi: 10.1158/1535-7163.MCT-19-0929. Mol Cancer Ther. 2020. PMID: 33008831 Free PMC article. Review.
-
Glioma‑neuronal interactions in tumor progression: Mechanism, therapeutic strategies and perspectives (Review).Int J Oncol. 2022 Sep;61(3):104. doi: 10.3892/ijo.2022.5394. Epub 2022 Jul 20. Int J Oncol. 2022. PMID: 35856439 Free PMC article. Review.
-
Challenges in the Therapeutic Targeting of KCa Channels: From Basic Physiology to Clinical Applications.Int J Mol Sci. 2024 Mar 4;25(5):2965. doi: 10.3390/ijms25052965. Int J Mol Sci. 2024. PMID: 38474212 Free PMC article. Review.
References
Publication types
MeSH terms
Substances
LinkOut - more resources
Full Text Sources
Miscellaneous