SLO potassium channels antagonize premature decision making in C. elegans
- PMID: 30272003
- PMCID: PMC6123717
- DOI: 10.1038/s42003-018-0124-5
SLO potassium channels antagonize premature decision making in C. elegans
Abstract
Animals must modify their behavior with appropriate timing to respond to environmental changes. Yet, the molecular and neural mechanisms regulating the timing of behavioral transition remain largely unknown. By performing forward genetics to reveal mechanisms that underlie the plasticity of thermotaxis behavior in C. elegans, we demonstrated that SLO potassium channels and a cyclic nucleotide-gated channel, CNG-3, determine the timing of transition of temperature preference after a shift in cultivation temperature. We further revealed that SLO and CNG-3 channels act in thermosensory neurons and decelerate alteration in the responsiveness of these neurons, which occurs prior to the preference transition after a temperature shift. Our results suggest that regulation of sensory adaptation is a major determinant of latency before animals make decisions to change their behavior.
Conflict of interest statement
The authors declare no competing interests.
Figures
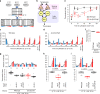
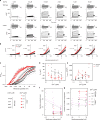
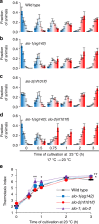
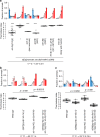
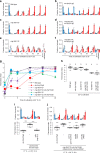
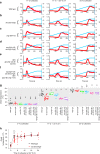
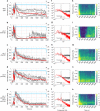

Similar articles
-
Environmental-temperature and internal-state dependent thermotaxis plasticity of nematodes.Curr Opin Neurobiol. 2022 Jun;74:102541. doi: 10.1016/j.conb.2022.102541. Epub 2022 Apr 18. Curr Opin Neurobiol. 2022. PMID: 35447377 Review.
-
Temperature- and touch-sensitive neurons couple CNG and TRPV channel activities to control heat avoidance in Caenorhabditis elegans.PLoS One. 2012;7(3):e32360. doi: 10.1371/journal.pone.0032360. Epub 2012 Mar 20. PLoS One. 2012. PMID: 22448218 Free PMC article.
-
Identification of guanylyl cyclases that function in thermosensory neurons of Caenorhabditis elegans.Genetics. 2006 Apr;172(4):2239-52. doi: 10.1534/genetics.105.050013. Epub 2006 Jan 16. Genetics. 2006. PMID: 16415369 Free PMC article.
-
The cyclic nucleotide gated channel subunit CNG-1 instructs behavioral outputs in Caenorhabditis elegans by coincidence detection of nutritional status and olfactory input.Neurosci Lett. 2016 Oct 6;632:71-8. doi: 10.1016/j.neulet.2016.08.037. Epub 2016 Aug 22. Neurosci Lett. 2016. PMID: 27561605
-
Cyclic nucleotide-gated ion channels.Physiol Rev. 2002 Jul;82(3):769-824. doi: 10.1152/physrev.00008.2002. Physiol Rev. 2002. PMID: 12087135 Review.
Cited by
-
cGMP dynamics that underlies thermosensation in temperature-sensing neuron regulates thermotaxis behavior in C. elegans.PLoS One. 2022 Dec 6;17(12):e0278343. doi: 10.1371/journal.pone.0278343. eCollection 2022. PLoS One. 2022. PMID: 36472979 Free PMC article.
-
Human FAM3C restores memory-based thermotaxis of Caenorhabditis elegans famp-1/m70.4 loss-of-function mutants.PNAS Nexus. 2022 Oct 25;1(5):pgac242. doi: 10.1093/pnasnexus/pgac242. eCollection 2022 Nov. PNAS Nexus. 2022. PMID: 36712359 Free PMC article.
-
How Caenorhabditis elegans Senses Mechanical Stress, Temperature, and Other Physical Stimuli.Genetics. 2019 May;212(1):25-51. doi: 10.1534/genetics.118.300241. Genetics. 2019. PMID: 31053616 Free PMC article. Review.
-
Mitochondrial ion channels in cardiac function.Am J Physiol Cell Physiol. 2021 Nov 1;321(5):C812-C825. doi: 10.1152/ajpcell.00246.2021. Epub 2021 Sep 22. Am J Physiol Cell Physiol. 2021. PMID: 34550794 Free PMC article. Review.
-
Feedforward and feedback mechanisms cooperatively regulate rapid experience-dependent response adaptation in a single thermosensory neuron type.bioRxiv [Preprint]. 2023 Dec 6:2023.12.05.570166. doi: 10.1101/2023.12.05.570166. bioRxiv. 2023. Update in: Proc Natl Acad Sci U S A. 2024 Apr 2;121(14):e2321430121. doi: 10.1073/pnas.2321430121 PMID: 38168209 Free PMC article. Updated. Preprint.
References
-
- Wang, X.-J. in Neuroeconomics 2nd edn (eds Glimcher, P. W. & Fehr, E.) Ch. 23 (Elsevier, 2014).
-
- Williams CT, Barnes BM, Kenagy GJ, Buck CL. Phenology of hibernation and reproduction in ground squirrels: integration of environmental cues with endogenous programming. J. Zool. 2014;292:112–124. doi: 10.1111/jzo.12103. - DOI
Grants and funding
LinkOut - more resources
Full Text Sources
Research Materials