A Shoot Fe Signaling Pathway Requiring the OPT3 Transporter Controls GSNO Reductase and Ethylene in Arabidopsis thaliana Roots
- PMID: 30254659
- PMCID: PMC6142016
- DOI: 10.3389/fpls.2018.01325
A Shoot Fe Signaling Pathway Requiring the OPT3 Transporter Controls GSNO Reductase and Ethylene in Arabidopsis thaliana Roots
Abstract
Ethylene, nitric oxide (NO) and glutathione (GSH) increase in Fe-deficient roots of Strategy I species where they participate in the up-regulation of Fe acquisition genes. However, S-nitrosoglutathione (GSNO), derived from NO and GSH, decreases in Fe-deficient roots. GSNO content is regulated by the GSNO-degrading enzyme S-nitrosoglutathione reductase (GSNOR). On the other hand, there are several results showing that the regulation of Fe acquisition genes does not solely depend on hormones and signaling molecules (such as ethylene or NO), which would act as activators, but also on the internal Fe content of plants, which would act as a repressor. Moreover, different results suggest that total Fe in roots is not the repressor of Fe acquisition genes, but rather the repressor is a Fe signal that moves from shoots to roots through the phloem [hereafter named LOng Distance Iron Signal (LODIS)]. To look further in the possible interactions between LODIS, ethylene and GSNOR, we compared Arabidopsis WT Columbia and LODIS-deficient mutant opt3-2 plants subjected to different Fe treatments that alter LODIS content. The opt3-2 mutant is impaired in the loading of shoot Fe into the phloem and presents constitutive expression of Fe acquisition genes. In roots of both Columbia and opt3-2 plants we determined 1-aminocyclopropane-1-carboxylic acid (ACC, ethylene precursor), expression of ethylene synthesis and signaling genes, and GSNOR expression and activity. The results obtained showed that both 'ethylene' (ACC and the expression of ethylene synthesis and signaling genes) and 'GSNOR' (expression and activity) increased in Fe-deficient WT Columbia roots. Additionally, Fe-sufficient opt3-2 roots had higher 'ethylene' and 'GSNOR' than Fe-sufficient WT Columbia roots. The increase of both 'ethylene' and 'GSNOR' was not related to the total root Fe content but to the absence of a Fe shoot signal (LODIS), and was associated with the up-regulation of Fe acquisition genes. The possible relationship between GSNOR(GSNO) and ethylene is discussed.
Keywords: S-nitrosoglutathione (GSNO); S-nitrosoglutathione reductase (GSNOR); ethylene; glutathione (GSH); iron; long distance iron signal (LODIS); nitric oxide (NO); phloem.
Figures
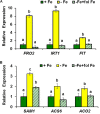
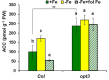
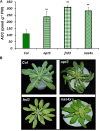
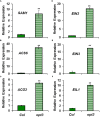
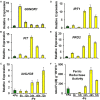
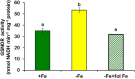
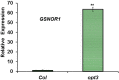
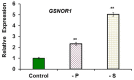
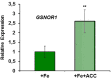
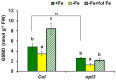
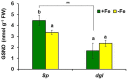
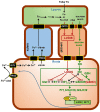
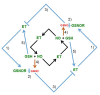
Similar articles
-
NO Is Not the Same as GSNO in the Regulation of Fe Deficiency Responses by Dicot Plants.Int J Mol Sci. 2023 Aug 9;24(16):12617. doi: 10.3390/ijms241612617. Int J Mol Sci. 2023. PMID: 37628796 Free PMC article. Review.
-
A shoot derived long distance iron signal may act upstream of the IMA peptides in the regulation of Fe deficiency responses in Arabidopsis thaliana roots.Front Plant Sci. 2022 Aug 29;13:971773. doi: 10.3389/fpls.2022.971773. eCollection 2022. Front Plant Sci. 2022. PMID: 36105702 Free PMC article.
-
Shoot to root communication is necessary to control the expression of iron-acquisition genes in Strategy I plants.Planta. 2013 Jan;237(1):65-75. doi: 10.1007/s00425-012-1757-0. Epub 2012 Sep 15. Planta. 2013. PMID: 22983673
-
A new model involving ethylene, nitric oxide and Fe to explain the regulation of Fe-acquisition genes in Strategy I plants.Plant Physiol Biochem. 2011 May;49(5):537-44. doi: 10.1016/j.plaphy.2011.01.019. Epub 2011 Jan 21. Plant Physiol Biochem. 2011. PMID: 21316254
-
Ethylene and Nitric Oxide Involvement in the Regulation of Fe and P Deficiency Responses in Dicotyledonous Plants.Int J Mol Sci. 2021 May 5;22(9):4904. doi: 10.3390/ijms22094904. Int J Mol Sci. 2021. PMID: 34063156 Free PMC article. Review.
Cited by
-
Chelators of iron and their role in plant's iron management.Physiol Mol Biol Plants. 2020 Aug;26(8):1541-1549. doi: 10.1007/s12298-020-00841-y. Epub 2020 Jul 7. Physiol Mol Biol Plants. 2020. PMID: 32801485 Free PMC article. Review.
-
NO Is Not the Same as GSNO in the Regulation of Fe Deficiency Responses by Dicot Plants.Int J Mol Sci. 2023 Aug 9;24(16):12617. doi: 10.3390/ijms241612617. Int J Mol Sci. 2023. PMID: 37628796 Free PMC article. Review.
-
Comparative Study of Several Fe Deficiency Responses in the Arabidopsis thaliana Ethylene Insensitive Mutants ein2-1 and ein2-5.Plants (Basel). 2021 Jan 29;10(2):262. doi: 10.3390/plants10020262. Plants (Basel). 2021. PMID: 33573082 Free PMC article.
-
Influence of Ethylene Signaling in the Crosstalk Between Fe, S, and P Deficiency Responses in Arabidopsis thaliana.Front Plant Sci. 2021 Mar 30;12:643585. doi: 10.3389/fpls.2021.643585. eCollection 2021. Front Plant Sci. 2021. PMID: 33859661 Free PMC article.
-
Micrografting Provides Evidence for Systemic Regulation of Sulfur Metabolism between Shoot and Root.Plants (Basel). 2021 Aug 20;10(8):1729. doi: 10.3390/plants10081729. Plants (Basel). 2021. PMID: 34451773 Free PMC article.
References
-
- Airaki M., Leterrier M., Mateos R. M., Valderrama R., Chaki M., Barroso J. B., et al. (2012). Metabolism of reactive oxygen species and reactive nitrogen species in pepper (Capsicum annuum L.) plants under low temperature stress. Plant Cell Environ. 35 281–295. 10.1111/j.1365-3040.2011.02310.x - DOI - PubMed
-
- Akmakjian G. Z. (2011). Long-Distance Cadmium Transport and Regulation of Heavy Metal Stress Responses in Arabidopsis thaliana. Master Science thesis, University of California; San Diego, CA.
-
- Alcántara E., Romera F. J., De La Guardia M. D., Cañete M. (1994). Effects of heavy metals on both induction and function of root Fe(III) reductase in Fe-deficient cucumber (Cucumis sativus L.) plants. J. Exp. Bot. 281 1893–1898. 10.1093/jxb/45.12.1893 - DOI
-
- Bacaicoa E., Mora V., Zamarreño A. M., Fuentes M., Casanova E., García-Mina J. M. (2011). Auxin: a major player in the shoot-to-root regulation of root Fe-stress physiological responses to Fe deficiency in cucumber plants. Plant Physiol. Biochem. 49 545–556. 10.1016/j.plaphy.2011.02.018 - DOI - PubMed
LinkOut - more resources
Full Text Sources
Other Literature Sources
Molecular Biology Databases