Coordinated Hibernation of Transcriptional and Translational Apparatus during Growth Transition of Escherichia coli to Stationary Phase
- PMID: 30225374
- PMCID: PMC6134199
- DOI: 10.1128/mSystems.00057-18
Coordinated Hibernation of Transcriptional and Translational Apparatus during Growth Transition of Escherichia coli to Stationary Phase
Abstract
In the process of Escherichia coli K-12 growth from exponential phase to stationary, marked alteration takes place in the pattern of overall genome expression through modulation of both parts of the transcriptional and translational apparatus. In transcription, the sigma subunit with promoter recognition properties is replaced from the growth-related factor RpoD by the stationary-phase-specific factor RpoS. The unused RpoD is stored by binding with the anti-sigma factor Rsd. In translation, the functional 70S ribosome is converted to inactive 100S dimers through binding with the ribosome modulation factor (RMF). Up to the present time, the regulatory mechanisms of expression of these two critical proteins, Rsd and RMF, have remained totally unsolved. In this study, attempts were made to identify the whole set of transcription factors involved in transcription regulation of the rsd and rmf genes using the newly developed promoter-specific transcription factor (PS-TF) screening system. In the first screening, 74 candidate TFs with binding activity to both of the rsd and rmf promoters were selected from a total of 194 purified TFs. After 6 cycles of screening, we selected 5 stress response TFs, ArcA, McbR, RcdA, SdiA, and SlyA, for detailed analysis in vitro and in vivo of their regulatory roles. Results indicated that both rsd and rmf promoters are repressed by ArcA and activated by McbR, RcdA, SdiA, and SlyA. We propose the involvement of a number of TFs in simultaneous and coordinated regulation of the transcriptional and translational apparatus. By using genomic SELEX (gSELEX) screening, each of the five TFs was found to regulate not only the rsd and rmf genes but also a variety of genes for growth and survival. IMPORTANCE During the growth transition of E. coli from exponential phase to stationary, the genome expression pattern is altered markedly. For this alteration, the transcription apparatus is altered by binding of anti-sigma factor Rsd to the RpoD sigma factor for sigma factor replacement, while the translation machinery is modulated by binding of RMF to 70S ribosome to form inactive ribosome dimer. Using the PS-TF screening system, a number of TFs were found to bind to both the rsd and rmf promoters, of which the regulatory roles of 5 representative TFs (one repressor ArcA and the four activators McbR, RcdA, SdiA, and SlyA) were analyzed in detail. The results altogether indicated the involvement of a common set of TFs, each sensing a specific environmental condition, in coordinated hibernation of the transcriptional and translational apparatus for adaptation and survival under stress conditions.
Keywords: Escherichia coli; PS-TF screening; anti-sigma factor Rsd; ribosome modulation factor RMF; stationary-phase adaptation.
Figures
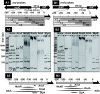
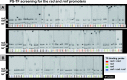
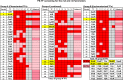
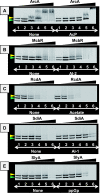
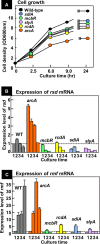
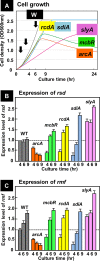
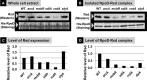
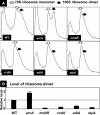
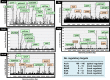
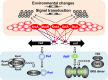
Similar articles
-
Coordinated Regulation of Rsd and RMF for Simultaneous Hibernation of Transcription Apparatus and Translation Machinery in Stationary-Phase Escherichia coli.Front Genet. 2019 Dec 4;10:1153. doi: 10.3389/fgene.2019.01153. eCollection 2019. Front Genet. 2019. PMID: 31867037 Free PMC article. Review.
-
Metal-Responsive Transcription Factors Co-Regulate Anti-Sigma Factor (Rsd) and Ribosome Dimerization Factor Expression.Int J Mol Sci. 2023 Mar 1;24(5):4717. doi: 10.3390/ijms24054717. Int J Mol Sci. 2023. PMID: 36902154 Free PMC article.
-
Modulation of the nucleoid, the transcription apparatus, and the translation machinery in bacteria for stationary phase survival.Genes Cells. 1999 Mar;4(3):135-43. doi: 10.1046/j.1365-2443.1999.00247.x. Genes Cells. 1999. PMID: 10320479 Review.
-
Expression of ribosome modulation factor (RMF) in Escherichia coli requires ppGpp.Genes Cells. 2001 Aug;6(8):665-76. doi: 10.1046/j.1365-2443.2001.00457.x. Genes Cells. 2001. PMID: 11532026
-
Regulation of the Escherichia coli rmf gene encoding the ribosome modulation factor: growth phase- and growth rate-dependent control.EMBO J. 1993 Feb;12(2):625-30. doi: 10.1002/j.1460-2075.1993.tb05695.x. EMBO J. 1993. PMID: 8440252 Free PMC article.
Cited by
-
Genome-Wide Mapping of the Escherichia coli PhoB Regulon Reveals Many Transcriptionally Inert, Intragenic Binding Sites.mBio. 2023 Jun 27;14(3):e0253522. doi: 10.1128/mbio.02535-22. Epub 2023 Apr 17. mBio. 2023. PMID: 37067422 Free PMC article.
-
Functional Sites of Ribosome Modulation Factor (RMF) Involved in the Formation of 100S Ribosome.Front Mol Biosci. 2021 May 3;8:661691. doi: 10.3389/fmolb.2021.661691. eCollection 2021. Front Mol Biosci. 2021. PMID: 34012979 Free PMC article.
-
Single-Target Regulators Constitute the Minority Group of Transcription Factors in Escherichia coli K-12.Front Microbiol. 2021 Jun 18;12:697803. doi: 10.3389/fmicb.2021.697803. eCollection 2021. Front Microbiol. 2021. PMID: 34220787 Free PMC article.
-
Trouble is coming: Signaling pathways that regulate general stress responses in bacteria.J Biol Chem. 2019 Aug 2;294(31):11685-11700. doi: 10.1074/jbc.REV119.005593. Epub 2019 Jun 13. J Biol Chem. 2019. PMID: 31197038 Free PMC article. Review.
-
Forming and waking dormant cells: The ppGpp ribosome dimerization persister model.Biofilm. 2020 Jan 7;2:100018. doi: 10.1016/j.bioflm.2019.100018. eCollection 2020 Dec. Biofilm. 2020. PMID: 33447804 Free PMC article.
References
-
- Bremer H, Dennis PP. 1996. Modulation of chemical composition and other parameters of the cell by growth rate, p 1553–1569. In Neidhardt JC, Curtiss R, III, Ingraham JL, Lin ECC, Low KB, Magasanik B, Reznikoff WS, Riley M, Schaechter M, Umbarger HE (ed), Escherichia coli and Salmonella typhimurium: cellular and molecular biology, 2nd ed, vol 2 ASM Press, Washington, DC.
-
- Keener J, Nomura M. 1996. Regulation of ribosome synthesis, p 1417–1431. In Neidhardt JC, Curtiss R, III, Ingraham JL, Lin ECC, Low KB, Magasanik B, Reznikoff WS, Riley M, Schaechter M, Umbarger HE (ed), Escherichia coli and Salmonella typhimurium: cellular and molecular biology, 2nd ed, vol 1 ASM Press, Washington, DC.
LinkOut - more resources
Full Text Sources
Other Literature Sources
Molecular Biology Databases
Research Materials
Miscellaneous