Rapid sample delivery for megahertz serial crystallography at X-ray FELs
- PMID: 30224961
- PMCID: PMC6126653
- DOI: 10.1107/S2052252518008369
Rapid sample delivery for megahertz serial crystallography at X-ray FELs
Abstract
Liquid microjets are a common means of delivering protein crystals to the focus of X-ray free-electron lasers (FELs) for serial femtosecond crystallography measurements. The high X-ray intensity in the focus initiates an explosion of the microjet and sample. With the advent of X-ray FELs with megahertz rates, the typical velocities of these jets must be increased significantly in order to replenish the damaged material in time for the subsequent measurement with the next X-ray pulse. This work reports the results of a megahertz serial diffraction experiment at the FLASH FEL facility using 4.3 nm radiation. The operation of gas-dynamic nozzles that produce liquid microjets with velocities greater than 80 m s-1 was demonstrated. Furthermore, this article provides optical images of X-ray-induced explosions together with Bragg diffraction from protein microcrystals exposed to trains of X-ray pulses repeating at rates of up to 4.5 MHz. The results indicate the feasibility for megahertz serial crystallography measurements with hard X-rays and give guidance for the design of such experiments.
Keywords: FELs; X-ray FEL pulse trains; X-ray free-electron lasers; megahertz repetition rates.
Figures
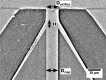
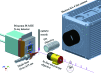
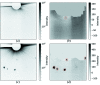
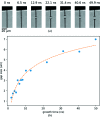
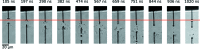
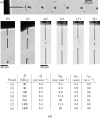
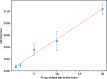
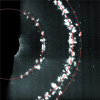
Similar articles
-
Megahertz pulse trains enable multi-hit serial femtosecond crystallography experiments at X-ray free electron lasers.Nat Commun. 2022 Aug 11;13(1):4708. doi: 10.1038/s41467-022-32434-6. Nat Commun. 2022. PMID: 35953469 Free PMC article.
-
Liquid sample delivery techniques for serial femtosecond crystallography.Philos Trans R Soc Lond B Biol Sci. 2014 Jul 17;369(1647):20130337. doi: 10.1098/rstb.2013.0337. Philos Trans R Soc Lond B Biol Sci. 2014. PMID: 24914163 Free PMC article. Review.
-
Megahertz data collection from protein microcrystals at an X-ray free-electron laser.Nat Commun. 2018 Aug 28;9(1):3487. doi: 10.1038/s41467-018-05953-4. Nat Commun. 2018. PMID: 30154468 Free PMC article.
-
Serial femtosecond crystallography: the first five years.IUCrJ. 2015 Feb 3;2(Pt 2):246-55. doi: 10.1107/S205225251402702X. eCollection 2015 Mar 1. IUCrJ. 2015. PMID: 25866661 Free PMC article. Review.
-
Emerging opportunities in structural biology with X-ray free-electron lasers.Curr Opin Struct Biol. 2012 Oct;22(5):613-26. doi: 10.1016/j.sbi.2012.07.015. Epub 2012 Aug 22. Curr Opin Struct Biol. 2012. PMID: 22922042 Free PMC article. Review.
Cited by
-
Recent trends in crystallography - a current IUCr journals perspective.IUCrJ. 2019 Nov 1;6(Pt 6):984-987. doi: 10.1107/S2052252519014507. eCollection 2019 Nov 1. IUCrJ. 2019. PMID: 31709052 Free PMC article.
-
Membrane protein megahertz crystallography at the European XFEL.Nat Commun. 2019 Nov 4;10(1):5021. doi: 10.1038/s41467-019-12955-3. Nat Commun. 2019. PMID: 31685819 Free PMC article.
-
Kilohertz droplet-on-demand serial femtosecond crystallography at the European XFEL station FXE.Struct Dyn. 2024 Apr 17;11(2):024310. doi: 10.1063/4.0000248. eCollection 2024 Mar. Struct Dyn. 2024. PMID: 38638699 Free PMC article.
-
Megahertz serial crystallography.Nat Commun. 2018 Oct 2;9(1):4025. doi: 10.1038/s41467-018-06156-7. Nat Commun. 2018. PMID: 30279492 Free PMC article.
-
High-throughput in situ experimental phasing.Acta Crystallogr D Struct Biol. 2020 Aug 1;76(Pt 8):790-801. doi: 10.1107/S2059798320009109. Epub 2020 Jul 28. Acta Crystallogr D Struct Biol. 2020. PMID: 32744261 Free PMC article.
References
-
- Acero, A. J., Ferrera, C., Montanero, J. M. & Gañán-Calvo, A. M. (2012). J. Micromech. Microeng. 22, 065011.
-
- Barends, T. R. M., Foucar, L., Ardevol, A., Nass, K., Aquila, A., Botha, S., Doak, R. B., Falahati, K., Hartmann, E., Hilpert, M., Heinz, M., Hoffmann, M. C., Köfinger, J., Koglin, J. E., Kovacsova, G., Liang, M., Milathianaki, D., Lemke, H. T., Reinstein, J., Roome, C. M., Shoeman, R. L., Williams, G. J., Burghardt, I., Hummer, G., Boutet, S. & Schlichting, I. (2015). Science, 350, 445–450. - PubMed
-
- Beyerlein, K. R., Adriano, L., Heymann, M., Kirian, R., Knoška, J., Wilde, F., Chapman, H. N. & Bajt, S. (2015). Rev. Sci. Instrum. 86, 125104. - PubMed
-
- Beyerlein, K. R., Dierksmeyer, D., Mariani, V., Kuhn, M., Sarrou, I., Ottaviano, A., Awel, S., Knoska, J., Fuglerud, S., Jönsson, O., Stern, S., Wiedorn, M. O., Yefanov, O., Adriano, L., Bean, R., Burkhardt, A., Fischer, P., Heymann, M., Horke, D. A., Jungnickel, K. E. J., Kovaleva, E., Lorbeer, O., Metz, M., Meyer, J., Morgan, A., Pande, K., Panneerselvam, S., Seuring, C., Tolstikova, A., Lieske, J., Aplin, S., Roessle, M., White, T. A., Chapman, H. N., Meents, A. & Oberthuer, D. (2017). IUCrJ, 4, 769–777. - PMC - PubMed
Grants and funding
LinkOut - more resources
Full Text Sources
Other Literature Sources