Flexibility of the Sec13/31 cage is influenced by the Sec31 C-terminal disordered domain
- PMID: 30172710
- PMCID: PMC6188663
- DOI: 10.1016/j.jsb.2018.08.016
Flexibility of the Sec13/31 cage is influenced by the Sec31 C-terminal disordered domain
Abstract
In COPII mediated vesicle formation, Sec13/Sec31 heterotetramers play a role in organizing the membranes into a spherical vesicle. There they oligomerize into a cage that interacts with the other COPII proteins to direct vesicle formation and concentrate cargo into a bud. In this role they must be flexible to accommodate different sizes and shapes of cargo, but also have elements that provide rigidity to help deform the membrane. Here we characterize the influence the C-terminal disordered region of Sec31 has on cage flexibility and rigidity. After deleting this region (residues 820-1220), we characterized Sec13/Sec31ΔC heterotetramers biophysically and structurally through cryo-EM. Our results show that Sec13/31ΔC self-assembles into canonical cuboctahedral cages in vitro at buffer conditions similar to wild type. The distribution of cage sizes indicated that unlike the wild type, Sec13/31ΔC cages have a more homogeneous geometry. However, the structure of cuboctahedrons exhibited more conformational heterogeneity than wild type. Through localized reconstruction of cage vertices and molecular dynamics flexible fitting we found a new hinge for the flexing of Sec31 β-propeller domain and more flexibility of the previously known hinge. Together, these results show that the C-terminal region of Sec31 regulates the flexing of other domains such that flexibility and rigidity are not compromised during transport of large and/or asymmetric cargo.
Keywords: Coat protein complex; Cryo-EM; Membrane remodeling; Subparticle refinement; Vesicle trafficking.
Copyright © 2018 Elsevier Inc. All rights reserved.
Figures
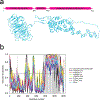
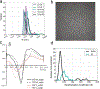
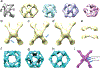
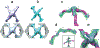
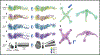
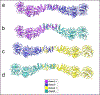
Similar articles
-
Structural basis for cargo regulation of COPII coat assembly.Cell. 2008 Aug 8;134(3):474-84. doi: 10.1016/j.cell.2008.06.024. Cell. 2008. PMID: 18692470 Free PMC article.
-
A pseudoatomic model of the COPII cage obtained from cryo-electron microscopy and mass spectrometry.Nat Struct Mol Biol. 2013 Feb;20(2):167-73. doi: 10.1038/nsmb.2467. Epub 2012 Dec 23. Nat Struct Mol Biol. 2013. PMID: 23262493 Free PMC article.
-
Structure of the Sec13/31 COPII coat cage.Nature. 2006 Jan 12;439(7073):234-8. doi: 10.1038/nature04339. Nature. 2006. PMID: 16407955
-
The highly conserved COPII coat complex sorts cargo from the endoplasmic reticulum and targets it to the golgi.Cold Spring Harb Perspect Biol. 2013 Feb 1;5(2):a013367. doi: 10.1101/cshperspect.a013367. Cold Spring Harb Perspect Biol. 2013. PMID: 23378591 Free PMC article. Review.
-
Making COPII coats.Cell. 2007 Jun 29;129(7):1251-2. doi: 10.1016/j.cell.2007.06.015. Cell. 2007. PMID: 17604713 Review.
Cited by
-
ER exit in physiology and disease.Front Mol Biosci. 2024 Jan 18;11:1352970. doi: 10.3389/fmolb.2024.1352970. eCollection 2024. Front Mol Biosci. 2024. PMID: 38314136 Free PMC article. Review.
-
COPII-mediated trafficking at the ER/ERGIC interface.Traffic. 2019 Jul;20(7):491-503. doi: 10.1111/tra.12654. Epub 2019 May 30. Traffic. 2019. PMID: 31059169 Free PMC article. Review.
-
COPII cage assembly factor Sec13 integrates information flow regulating endomembrane function in response to human variation.Sci Rep. 2024 May 3;14(1):10160. doi: 10.1038/s41598-024-60687-2. Sci Rep. 2024. PMID: 38698045 Free PMC article.
-
Probing intracellular vesicle trafficking and membrane remodelling by cryo-EM.J Struct Biol. 2022 Mar;214(1):107836. doi: 10.1016/j.jsb.2022.107836. Epub 2022 Jan 31. J Struct Biol. 2022. PMID: 35101600 Free PMC article. Review.
-
Structure of the complete, membrane-assembled COPII coat reveals a complex interaction network.Nat Commun. 2021 Apr 1;12(1):2034. doi: 10.1038/s41467-021-22110-6. Nat Commun. 2021. PMID: 33795673 Free PMC article.
References
Publication types
MeSH terms
Substances
Grants and funding
LinkOut - more resources
Full Text Sources
Other Literature Sources