The plasticity of primary microglia and their multifaceted effects on endogenous neural stem cells in vitro and in vivo
- PMID: 30103769
- PMCID: PMC6090672
- DOI: 10.1186/s12974-018-1261-y
The plasticity of primary microglia and their multifaceted effects on endogenous neural stem cells in vitro and in vivo
Abstract
Background: Microglia-the resident immune cells of the brain-are activated after brain lesions, e.g., cerebral ischemia, and polarize towards a classic "M1" pro-inflammatory or an alternative "M2" anti-inflammatory phenotype following characteristic temporo-spatial patterns, contributing either to secondary tissue damage or to regenerative responses. They closely interact with endogenous neural stem cells (NSCs) residing in distinct niches of the adult brain. The current study aimed at elucidating the dynamics of microglia polarization and their differential effects on NSC function.
Results: Primary rat microglia in vitro were polarized towards a M1 phenotype by LPS, or to a M2 phenotype by IL4, while simultaneous exposure to LPS plus IL4 resulted in a hybrid phenotype expressing both M1- and M2-characteristic markers. M2 microglia migrated less but exhibit higher phagocytic activity than M1 microglia. Defined mediators switched microglia from one polarization state to the other, a process more effective when transforming M2 microglia towards M1 than vice versa. Polarized microglia had differential effects on the differentiation potential of NSCs in vitro and in vivo, with M1 microglia promoting astrocytogenesis, while M2 microglia supported neurogenesis. Regardless of their polarization, microglia inhibited NSC proliferation, increased NSC migration, and accelerated NSC differentiation.
Conclusion: Overall, this study shed light on the complex conditions governing microglia polarization and the effects of differentially polarized microglia on critical functions of NSCs in vitro and in vivo. Refining the understanding of microglia activation and their modulatory effects on NSCs is likely to facilitate the development of innovative therapeutic concepts supporting the innate regenerative capacity of the brain.
Keywords: Cerebral ischemia; Hybrid microglia; M1 microglia; M2 microglia; Neuroinflammation; Neuroprotection; Stem cell-mediated regeneration.
Conflict of interest statement
All animal procedures for tissue harvesting as well as for in vivo experiments were in accordance with the German Laws for Animal Protection. The study was approved by the local animal care committee (Tierschutz-Beauftragte University of Cologne) and local governmental authorities (Landesamt für Natur, Umwelt und Verbraucherschutz North Rhine-Westphalia, LANUV NRW, AZ 84-02.05.40.14.056, AZ UniKoeln_Anzeige§4.16.020 and AZ 84-02.04.2012.A116).
Not applicable
The authors declare that they have no competing interests.
Springer Nature remains neutral with regard to jurisdictional claims in published maps and institutional affiliations.
Figures
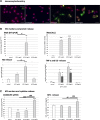
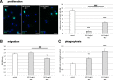
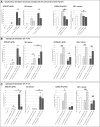
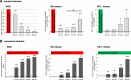
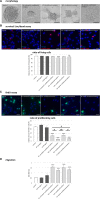
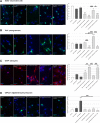
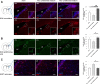
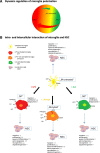
Similar articles
-
Cyclic AMP is a key regulator of M1 to M2a phenotypic conversion of microglia in the presence of Th2 cytokines.J Neuroinflammation. 2016 Jan 13;13:9. doi: 10.1186/s12974-015-0463-9. J Neuroinflammation. 2016. PMID: 26757726 Free PMC article.
-
The Secretome of Microglia Regulate Neural Stem Cell Function.Neuroscience. 2019 May 1;405:92-102. doi: 10.1016/j.neuroscience.2017.10.034. Epub 2017 Nov 1. Neuroscience. 2019. PMID: 29101080
-
Anti-inflammatory effects of anisalcohol on lipopolysaccharide-stimulated BV2 microglia via selective modulation of microglia polarization and down-regulation of NF-κB p65 and JNK activation.Mol Immunol. 2018 Mar;95:39-46. doi: 10.1016/j.molimm.2018.01.011. Epub 2018 Feb 20. Mol Immunol. 2018. PMID: 29407575
-
Immunopharmacological intervention for successful neural stem cell therapy: New perspectives in CNS neurogenesis and repair.Pharmacol Ther. 2014 Jan;141(1):21-31. doi: 10.1016/j.pharmthera.2013.08.001. Epub 2013 Aug 15. Pharmacol Ther. 2014. PMID: 23954656 Review.
-
Diversity and plasticity of microglial cells in psychiatric and neurological disorders.Pharmacol Ther. 2015 Oct;154:21-35. doi: 10.1016/j.pharmthera.2015.06.010. Epub 2015 Jun 27. Pharmacol Ther. 2015. PMID: 26129625 Review.
Cited by
-
Low-Dose Ionizing Radiation Modulates Microglia Phenotypes in the Models of Alzheimer's Disease.Int J Mol Sci. 2020 Jun 25;21(12):4532. doi: 10.3390/ijms21124532. Int J Mol Sci. 2020. PMID: 32630597 Free PMC article.
-
Developmental dynamics of neurogenesis and gliogenesis in the postnatal mammalian brain in health and disease: Historical and future perspectives.Wiley Interdiscip Rev Dev Biol. 2020 May;9(3):e369. doi: 10.1002/wdev.369. Epub 2019 Dec 11. Wiley Interdiscip Rev Dev Biol. 2020. PMID: 31825170 Free PMC article. Review.
-
Modulation of microglial polarization by sequential targeting surface-engineered exosomes improves therapy for ischemic stroke.Drug Deliv Transl Res. 2024 Feb;14(2):418-432. doi: 10.1007/s13346-023-01408-6. Epub 2023 Aug 16. Drug Deliv Transl Res. 2024. PMID: 37587291
-
Microglia subtypes show substrate- and time-dependent phagocytosis preferences and phenotype plasticity.Front Immunol. 2022 Aug 29;13:945485. doi: 10.3389/fimmu.2022.945485. eCollection 2022. Front Immunol. 2022. PMID: 36105813 Free PMC article.
-
New Mechanistic Insights, Novel Treatment Paradigms, and Clinical Progress in Cerebrovascular Diseases.Front Aging Neurosci. 2021 Jan 28;13:623751. doi: 10.3389/fnagi.2021.623751. eCollection 2021. Front Aging Neurosci. 2021. PMID: 33584250 Free PMC article.
References
-
- Walberer M, Jantzen SU, Backes H, Rueger MA, Keuters MH, Neumaier B, Hoehn M, Fink GR, Graf R, Schroeter M. In-vivo detection of inflammation and neurodegeneration in the chronic phase after permanent embolic stroke in rats. Brain Res. 2014;1581:80–88. doi: 10.1016/j.brainres.2014.05.030. - DOI - PubMed
MeSH terms
Substances
Grants and funding
LinkOut - more resources
Full Text Sources
Other Literature Sources
Miscellaneous