Exploring the role of stromal osmoregulation in cancer and disease using executable modelling
- PMID: 30069015
- PMCID: PMC6070494
- DOI: 10.1038/s41467-018-05414-y
Exploring the role of stromal osmoregulation in cancer and disease using executable modelling
Abstract
Osmotic regulation is a vital homoeostatic process in all cells and tissues. Cells initially respond to osmotic stresses by activating transmembrane transport proteins to move osmotically active ions. Disruption of ion and water transport is frequently observed in cellular transformations such as cancer. We report that genes involved in membrane transport are significantly deregulated in many cancers, and that their expression can distinguish cancer cells from normal cells with a high degree of accuracy. We present an executable model of osmotic regulation and membrane transport in mammalian cells, providing a mechanistic explanation for phenotype change in varied disease states, and accurately predicting behaviour from single cell expression data. We also predict key proteins involved in cellular transformation, SLC4A3 (AE3), and SLC9A1 (NHE1). Furthermore, we predict and verify a synergistic drug combination in vitro, of sodium and chloride channel inhibitors, which target the osmoregulatory network to reduce cancer-associated phenotypes in fibroblasts.
Conflict of interest statement
The authors declare no competing interests.
Figures
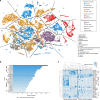
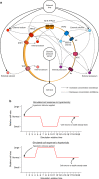
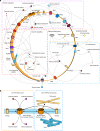
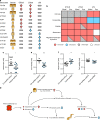
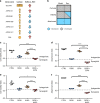
Similar articles
-
Lack of transforming growth factor-β signaling promotes collective cancer cell invasion through tumor-stromal crosstalk.Breast Cancer Res. 2012 Jul 2;14(4):R98. doi: 10.1186/bcr3217. Breast Cancer Res. 2012. PMID: 22748014 Free PMC article.
-
MicroRNA regulation in cancer-associated fibroblasts.Cancer Immunol Immunother. 2012 Feb;61(2):231-237. doi: 10.1007/s00262-011-1139-7. Epub 2011 Nov 16. Cancer Immunol Immunother. 2012. PMID: 22083346 Free PMC article. Review.
-
Arousal of cancer-associated stroma: overexpression of palladin activates fibroblasts to promote tumor invasion.PLoS One. 2012;7(1):e30219. doi: 10.1371/journal.pone.0030219. Epub 2012 Jan 23. PLoS One. 2012. PMID: 22291919 Free PMC article.
-
Elevated Na/H exchanger 1 (SLC9A1) emerges as a marker for tumorigenesis and prognosis in gliomas.J Exp Clin Cancer Res. 2018 Oct 17;37(1):255. doi: 10.1186/s13046-018-0923-z. J Exp Clin Cancer Res. 2018. PMID: 30333031 Free PMC article.
-
Chloride channels in cancer: Focus on chloride intracellular channel 1 and 4 (CLIC1 AND CLIC4) proteins in tumor development and as novel therapeutic targets.Biochim Biophys Acta. 2015 Oct;1848(10 Pt B):2523-31. doi: 10.1016/j.bbamem.2014.12.012. Epub 2014 Dec 26. Biochim Biophys Acta. 2015. PMID: 25546839 Review.
Cited by
-
MicroRNA-Mediated Metabolic Reprograming in Renal Cancer.Cancers (Basel). 2019 Nov 20;11(12):1825. doi: 10.3390/cancers11121825. Cancers (Basel). 2019. PMID: 31756931 Free PMC article.
-
Development of a 32-gene signature using machine learning for accurate prediction of inflammatory bowel disease.Cell Regen. 2023 Jan 5;12(1):8. doi: 10.1186/s13619-022-00143-6. Cell Regen. 2023. PMID: 36600111 Free PMC article.
-
Roles of hsa-miR-12462 and SLC9A1 in acute myeloid leukemia.J Hematol Oncol. 2020 Jul 23;13(1):101. doi: 10.1186/s13045-020-00935-w. J Hematol Oncol. 2020. PMID: 32703317 Free PMC article. Review.
-
Pump up the volume.Elife. 2024 Jul 2;13:e100032. doi: 10.7554/eLife.100032. Elife. 2024. PMID: 38953882 Free PMC article.
-
Potential Theranostic Roles of SLC4 Molecules in Human Diseases.Int J Mol Sci. 2023 Oct 13;24(20):15166. doi: 10.3390/ijms242015166. Int J Mol Sci. 2023. PMID: 37894847 Free PMC article. Review.
References
Publication types
MeSH terms
Grants and funding
LinkOut - more resources
Full Text Sources
Other Literature Sources
Miscellaneous