Evidence for Compartmentalized Axonal Mitochondrial Biogenesis: Mitochondrial DNA Replication Increases in Distal Axons As an Early Response to Parkinson's Disease-Relevant Stress
- PMID: 30030401
- PMCID: PMC6104298
- DOI: 10.1523/JNEUROSCI.0541-18.2018
Evidence for Compartmentalized Axonal Mitochondrial Biogenesis: Mitochondrial DNA Replication Increases in Distal Axons As an Early Response to Parkinson's Disease-Relevant Stress
Abstract
Dysregulation of mitochondrial biogenesis is implicated in the pathogenesis of neurodegenerative diseases such as Parkinson's disease (PD). However, it is not clear how mitochondrial biogenesis is regulated in neurons, with their unique compartmentalized anatomy and energetic demands. This is particularly relevant in PD because selectively vulnerable neurons feature long, highly arborized axons where degeneration initiates. We previously found that exposure of neurons to chronic, sublethal doses of rotenone, a complex I inhibitor linked to PD, causes early increases in mitochondrial density specifically in distal axons, suggesting possible upregulation of mitochondrial biogenesis within axons. Here, we directly evaluated for evidence of mitochondrial biogenesis in distal axons and examined whether PD-relevant stress causes compartmentalized alterations. Using BrdU labeling and imaging to quantify replicating mitochondrial DNA (mtDNA) in primary rat neurons (pooled from both sexes), we provide evidence of mtDNA replication in axons along with cell bodies and proximal dendrites. We found that exposure to chronic, sublethal rotenone increases mtDNA replication first in neurites and later extending to cell bodies, complementing our mitochondrial density data. Further, isolating axons from cell bodies and dendrites, we discovered that rotenone exposure upregulates mtDNA replication in distal axons. Utilizing superresolution stimulated emission depletion (STED) imaging, we identified mtDNA replication at sites of mitochondrial-endoplasmic reticulum contacts in axons. Our evidence suggests that mitochondrial biogenesis occurs not only in cell bodies, but also in distal axons, and is altered under PD-relevant stress conditions in an anatomically compartmentalized manner. We hypothesize that this contributes to vulnerability in neurodegenerative diseases.SIGNIFICANCE STATEMENT Mitochondrial biogenesis is crucial for maintaining mitochondrial and cellular health and has been linked to neurodegenerative disease pathogenesis. However, regulation of this process is poorly understood in CNS neurons, which rely on mitochondrial function for survival. Our findings offer fundamental insight into these regulatory mechanisms by demonstrating that replication of mitochondrial DNA, an essential precursor for biogenesis, can occur in distal regions of CNS neuron axons independent of the soma. Further, this process is upregulated specifically in axons as an early response to neurodegeneration-relevant stress. This is the first demonstration of the compartmentalized regulation of CNS neuronal mitochondrial biogenesis in response to stress and may prove a useful target in development of therapeutic strategies for neurodegenerative disease.
Keywords: Parkinson's disease; axonal; biogenesis; mitochondrial; neurodegeneration; rotenone.
Copyright © 2018 the authors 0270-6474/18/387506-11$15.00/0.
Figures
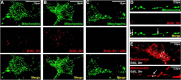
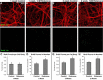
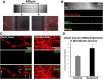
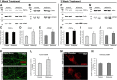
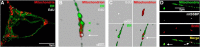
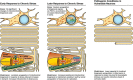
Similar articles
-
The Role of PGC-1α-Mediated Mitochondrial Biogenesis in Neurons.Neurochem Res. 2023 Sep;48(9):2595-2606. doi: 10.1007/s11064-023-03934-8. Epub 2023 Apr 25. Neurochem Res. 2023. PMID: 37097395 Review.
-
Integrating multiple aspects of mitochondrial dynamics in neurons: age-related differences and dynamic changes in a chronic rotenone model.Neurobiol Dis. 2011 Jan;41(1):189-200. doi: 10.1016/j.nbd.2010.09.006. Epub 2010 Sep 17. Neurobiol Dis. 2011. PMID: 20850532 Free PMC article.
-
The Interaction of Mitochondrial Biogenesis and Fission/Fusion Mediated by PGC-1α Regulates Rotenone-Induced Dopaminergic Neurotoxicity.Mol Neurobiol. 2017 Jul;54(5):3783-3797. doi: 10.1007/s12035-016-9944-9. Epub 2016 Jun 7. Mol Neurobiol. 2017. PMID: 27271125
-
Resveratrol Regulates Mitochondrial Biogenesis and Fission/Fusion to Attenuate Rotenone-Induced Neurotoxicity.Oxid Med Cell Longev. 2016;2016:6705621. doi: 10.1155/2016/6705621. Epub 2015 Dec 6. Oxid Med Cell Longev. 2016. PMID: 26770656 Free PMC article.
-
Impaired mitochondrial dynamics and function in the pathogenesis of Parkinson's disease.Exp Neurol. 2009 Aug;218(2):235-46. doi: 10.1016/j.expneurol.2009.03.006. Epub 2009 Mar 18. Exp Neurol. 2009. PMID: 19303005 Review.
Cited by
-
TSG101 negatively regulates mitochondrial biogenesis in axons.Proc Natl Acad Sci U S A. 2021 May 18;118(20):e2018770118. doi: 10.1073/pnas.2018770118. Proc Natl Acad Sci U S A. 2021. PMID: 33972422 Free PMC article.
-
Programming axonal mitochondrial maintenance and bioenergetics in neurodegeneration and regeneration.Neuron. 2022 Jun 15;110(12):1899-1923. doi: 10.1016/j.neuron.2022.03.015. Epub 2022 Apr 16. Neuron. 2022. PMID: 35429433 Free PMC article. Review.
-
Mitochondrial DNA Transport in Drosophila Neurons.Methods Mol Biol. 2022;2431:409-416. doi: 10.1007/978-1-0716-1990-2_21. Methods Mol Biol. 2022. PMID: 35412289
-
Multi-compartment Microfluidic Device Geometry and Covalently Bound Poly-D-Lysine Influence Neuronal Maturation.Front Bioeng Biotechnol. 2019 May 7;7:84. doi: 10.3389/fbioe.2019.00084. eCollection 2019. Front Bioeng Biotechnol. 2019. PMID: 31134192 Free PMC article.
-
The Role of PGC-1α-Mediated Mitochondrial Biogenesis in Neurons.Neurochem Res. 2023 Sep;48(9):2595-2606. doi: 10.1007/s11064-023-03934-8. Epub 2023 Apr 25. Neurochem Res. 2023. PMID: 37097395 Review.
References
-
- Aschrafi A, Kar AN, Gale JR, Elkahloun AG, Vargas JN, Sales N, Wilson G, Tompkins M, Gioio AE, Kaplan BB (2016) A heterogeneous population of nuclear-encoded mitochondrial mRNAs is present in the axons of primary sympathetic neurons. Mitochondrion 30:18–23. 10.1016/j.mito.2016.06.002 - DOI - PMC - PubMed
Publication types
MeSH terms
Substances
Grants and funding
LinkOut - more resources
Full Text Sources
Other Literature Sources
Medical
Research Materials