A radical S-adenosyl-L-methionine enzyme and a methyltransferase catalyze cyclopropane formation in natural product biosynthesis
- PMID: 30018376
- PMCID: PMC6050322
- DOI: 10.1038/s41467-018-05217-1
A radical S-adenosyl-L-methionine enzyme and a methyltransferase catalyze cyclopropane formation in natural product biosynthesis
Abstract
Cyclopropanation of unactivated olefinic bonds via addition of a reactive one-carbon species is well developed in synthetic chemistry, whereas natural cyclopropane biosynthesis employing this strategy is very limited. Here, we identify a two-component cyclopropanase system, composed of a HemN-like radical S-adenosyl-L-methionine (SAM) enzyme C10P and a methyltransferase C10Q, catalyzes chemically challenging cyclopropanation in the antitumor antibiotic CC-1065 biosynthesis. C10P uses its [4Fe-4S] cluster for reductive cleavage of the first SAM to yield a highly reactive 5'-deoxyadenosyl radical, which abstracts a hydrogen from the second SAM to produce a SAM methylene radical that adds to an sp2-hybridized carbon of substrate to form a SAM-substrate adduct. C10Q converts this adduct to CC-1065 via an intramolecular SN2 cyclization mechanism with elimination of S-adenosylhomocysteine. This cyclopropanation strategy not only expands the enzymatic reactions catalyzed by the radical SAM enzymes and methyltransferases, but also sheds light on previously unnoticed aspects of the versatile SAM-based biochemistry.
Conflict of interest statement
The authors declare no competing interests.
Figures

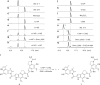
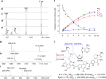
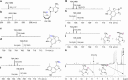
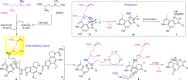
Similar articles
-
Crystallographic snapshots of sulfur insertion by lipoyl synthase.Proc Natl Acad Sci U S A. 2016 Aug 23;113(34):9446-50. doi: 10.1073/pnas.1602486113. Epub 2016 Aug 9. Proc Natl Acad Sci U S A. 2016. PMID: 27506792 Free PMC article.
-
Cfr and RlmN contain a single [4Fe-4S] cluster, which directs two distinct reactivities for S-adenosylmethionine: methyl transfer by SN2 displacement and radical generation.J Am Chem Soc. 2011 Dec 14;133(49):19586-9. doi: 10.1021/ja207327v. Epub 2011 Nov 18. J Am Chem Soc. 2011. PMID: 21916495 Free PMC article.
-
Auxiliary iron-sulfur cofactors in radical SAM enzymes.Biochim Biophys Acta. 2015 Jun;1853(6):1316-34. doi: 10.1016/j.bbamcr.2015.01.002. Epub 2015 Jan 15. Biochim Biophys Acta. 2015. PMID: 25597998 Review.
-
C-Methylation of S-adenosyl-L-Methionine Occurs Prior to Cyclopropanation in the Biosynthesis of 1-Amino-2-Methylcyclopropanecarboxylic Acid (Norcoronamic Acid) in a Bacterium.Biomolecules. 2020 May 16;10(5):775. doi: 10.3390/biom10050775. Biomolecules. 2020. PMID: 32429436 Free PMC article.
-
Identification and function of auxiliary iron-sulfur clusters in radical SAM enzymes.Biochim Biophys Acta. 2012 Nov;1824(11):1196-212. doi: 10.1016/j.bbapap.2012.07.009. Epub 2012 Jul 28. Biochim Biophys Acta. 2012. PMID: 22846545 Review.
Cited by
-
Impact of One-Carbon Metabolism-Driving Epitranscriptome as a Therapeutic Target for Gastrointestinal Cancer.Int J Mol Sci. 2021 Jul 6;22(14):7278. doi: 10.3390/ijms22147278. Int J Mol Sci. 2021. PMID: 34298902 Free PMC article. Review.
-
Functional Diversity of HemN-like Proteins.ACS Bio Med Chem Au. 2022 Jan 18;2(2):109-119. doi: 10.1021/acsbiomedchemau.1c00058. eCollection 2022 Apr 20. ACS Bio Med Chem Au. 2022. PMID: 37101745 Free PMC article. Review.
-
Diversity of the reaction mechanisms of SAM-dependent enzymes.Acta Pharm Sin B. 2021 Mar;11(3):632-650. doi: 10.1016/j.apsb.2020.08.011. Epub 2020 Aug 26. Acta Pharm Sin B. 2021. PMID: 33777672 Free PMC article. Review.
-
Biosynthesis of DNA-Alkylating Antitumor Natural Products.Molecules. 2022 Sep 27;27(19):6387. doi: 10.3390/molecules27196387. Molecules. 2022. PMID: 36234921 Free PMC article. Review.
-
Pathogenic bacteria remodel central metabolic enzyme to build a cyclopropanol warhead.Nat Chem. 2022 Aug;14(8):884-890. doi: 10.1038/s41557-022-01005-z. Epub 2022 Jul 29. Nat Chem. 2022. PMID: 35906404 Free PMC article.
References
Publication types
MeSH terms
Substances
Grants and funding
LinkOut - more resources
Full Text Sources
Other Literature Sources