PDGF-BB regulates splitting angiogenesis in skeletal muscle by limiting VEGF-induced endothelial proliferation
- PMID: 30014172
- PMCID: PMC6208885
- DOI: 10.1007/s10456-018-9634-5
PDGF-BB regulates splitting angiogenesis in skeletal muscle by limiting VEGF-induced endothelial proliferation
Abstract
VEGF induces normal or aberrant angiogenesis depending on its dose in the microenvironment around each producing cell in vivo. This transition depends on the balance between VEGF-induced endothelial stimulation and PDGF-BB-mediated pericyte recruitment, and co-expression of PDGF-BB normalizes aberrant angiogenesis despite high VEGF doses. We recently found that VEGF over-expression induces angiogenesis in skeletal muscle through an initial circumferential vascular enlargement followed by longitudinal splitting, rather than sprouting. Here we investigated the cellular mechanism by which PDGF-BB co-expression normalizes VEGF-induced aberrant angiogenesis. Monoclonal populations of transduced myoblasts, expressing similarly high levels of VEGF alone or with PDGF-BB, were implanted in mouse skeletal muscles. PDGF-BB co-expression did not promote sprouting and angiogenesis that occurred through vascular enlargement and splitting. However, enlargements were significantly smaller in diameter, due to a significant reduction in endothelial proliferation, and retained pericytes, which were otherwise lost with high VEGF alone. A time-course of histological analyses and repetitive intravital imaging showed that PDGF-BB co-expression anticipated the initiation of vascular enlargement and markedly accelerated the splitting process. Interestingly, quantification during in vivo imaging suggested that a global reduction in shear stress favored the initiation of transluminal pillar formation during VEGF-induced splitting angiogenesis. Quantification of target gene expression showed that VEGF-R2 signaling output was significantly reduced by PDGF-BB co-expression compared to VEGF alone. In conclusion, PDGF-BB co-expression prevents VEGF-induced aberrant angiogenesis by modulating VEGF-R2 signaling and endothelial proliferation, thereby limiting the degree of circumferential enlargement and enabling efficient completion of vascular splitting into normal capillary networks despite high VEGF doses.
Keywords: Intussusception; PDGF-BB; Pericytes; Shear stress; VEGF; Vascular splitting.
Conflict of interest statement
Conflict of interest
The authors declare that they have no conflict of interest.
Ethical standards
All procedures performed in studies involving animals were in accordance with the ethical standards of the Institutions at which the studies were conducted and were approved by the local Swiss and German Animal Welfare Committees.
Figures
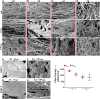
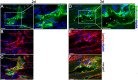
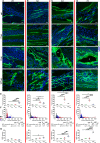
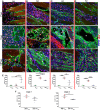
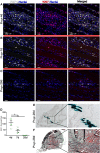
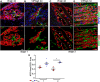
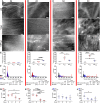
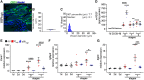
Similar articles
-
VEGF over-expression in skeletal muscle induces angiogenesis by intussusception rather than sprouting.Angiogenesis. 2013 Jan;16(1):123-36. doi: 10.1007/s10456-012-9304-y. Epub 2012 Sep 9. Angiogenesis. 2013. PMID: 22961440
-
EphrinB2/EphB4 signaling regulates non-sprouting angiogenesis by VEGF.EMBO Rep. 2018 May;19(5):e45054. doi: 10.15252/embr.201745054. Epub 2018 Apr 11. EMBO Rep. 2018. PMID: 29643120 Free PMC article.
-
Long-term safety and stability of angiogenesis induced by balanced single-vector co-expression of PDGF-BB and VEGF164 in skeletal muscle.Sci Rep. 2016 Feb 17;6:21546. doi: 10.1038/srep21546. Sci Rep. 2016. PMID: 26882992 Free PMC article.
-
Split for the cure: VEGF, PDGF-BB and intussusception in therapeutic angiogenesis.Biochem Soc Trans. 2014 Dec;42(6):1637-42. doi: 10.1042/BST20140234. Biochem Soc Trans. 2014. PMID: 25399582 Review.
-
Modulation of physiological angiogenesis in skeletal muscle by mechanical forces: involvement of VEGF and metalloproteinases.Angiogenesis. 2003;6(1):1-14. doi: 10.1023/a:1025809808697. Angiogenesis. 2003. PMID: 14517399 Review.
Cited by
-
Histological characteristics of exercise-induced skeletal muscle remodelling.J Cell Mol Med. 2023 Nov;27(21):3217-3234. doi: 10.1111/jcmm.17879. Epub 2023 Jul 30. J Cell Mol Med. 2023. PMID: 37517049 Free PMC article.
-
PDGFR in PDGF-BB/PDGFR Signaling Pathway Does Orchestrates Osteogenesis in a Temporal Manner.Research (Wash D C). 2023 May 5;6:0086. doi: 10.34133/research.0086. eCollection 2023. Research (Wash D C). 2023. PMID: 37223474 Free PMC article.
-
A novel retinoic acid drug, EYE-502, inhibits choroidal neovascularization by targeting endothelial cells and pericytes.Sci Rep. 2023 Jun 27;13(1):10439. doi: 10.1038/s41598-023-37619-7. Sci Rep. 2023. PMID: 37369771 Free PMC article.
-
A Metabolic Signature to Monitor Endothelial Cell Differentiation, Activation, and Vascular Organization.Biomedicines. 2022 Sep 15;10(9):2293. doi: 10.3390/biomedicines10092293. Biomedicines. 2022. PMID: 36140393 Free PMC article.
-
HSPA12A Stimulates p38/ERK-AP-1 Signaling to Promote Angiogenesis and Is Required for Functional Recovery Postmyocardial Infarction.Oxid Med Cell Longev. 2022 Jun 22;2022:2333848. doi: 10.1155/2022/2333848. eCollection 2022. Oxid Med Cell Longev. 2022. PMID: 35783189 Free PMC article.
References
-
- Benjamin EJ, Virani SS, Callaway CW, Chang AR, Cheng S, Chiuve SE, Cushman M, Delling FN, Deo R, de Ferranti SD, Ferguson JF, Fornage M, Gillespie C, Isasi CR, Jimenez MC, Jordan LC, Judd SE, Lackland D, Lichtman JH, Lisabeth L, Liu S, Longenecker CT, Lutsey PL, Matchar DB, Matsushita K, Mussolino ME, Nasir K, O’Flaherty M, Palaniappan LP, Pandey DK, Reeves MJ, Ritchey MD, Rodriguez CJ, Roth GA, Rosamond WD, Sampson UKA, Satou GM, Shah SH, Spartano NL, Tirschwell DL, Tsao CW, Voeks JH, Willey JZ, Wilkins JT, Wu JH, Alger HM, Wong SS, Muntner P. Heart disease and stroke statistics-2018 update: a report from the American Heart Association. Circulation. 2018 doi: 10.1161/CIR.0000000000000558. - DOI - PubMed
Publication types
MeSH terms
Substances
Grants and funding
LinkOut - more resources
Full Text Sources
Other Literature Sources