Trapping of the transport-segment DNA by the ATPase domains of a type II topoisomerase
- PMID: 29968711
- PMCID: PMC6030046
- DOI: 10.1038/s41467-018-05005-x
Trapping of the transport-segment DNA by the ATPase domains of a type II topoisomerase
Abstract
Type II topoisomerases alter DNA topology to control DNA supercoiling and chromosome segregation and are targets of clinically important anti-infective and anticancer therapeutics. They act as ATP-operated clamps to trap a DNA helix and transport it through a transient break in a second DNA. Here, we present the first X-ray crystal structure solved at 2.83 Å of a closed clamp complete with trapped T-segment DNA obtained by co-crystallizing the ATPase domain of S. pneumoniae topoisomerase IV with a nonhydrolyzable ATP analogue and 14-mer duplex DNA. The ATPase dimer forms a 22 Å protein hole occupied by the kinked DNA bound asymmetrically through positively charged residues lining the hole, and whose mutagenesis impacts the DNA decatenation, DNA relaxation and DNA-dependent ATPase activities of topo IV. These results and a side-bound DNA-ParE structure help explain how the T-segment DNA is captured and transported by a type II topoisomerase, and reveal a new enzyme-DNA interface for drug discovery.
Conflict of interest statement
The authors declare no competing interests.
Figures
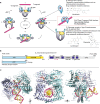
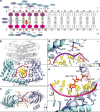
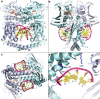
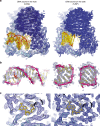
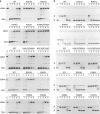
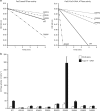
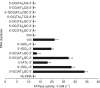
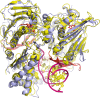
Similar articles
-
Structure of an 'open' clamp type II topoisomerase-DNA complex provides a mechanism for DNA capture and transport.Nucleic Acids Res. 2013 Nov;41(21):9911-23. doi: 10.1093/nar/gkt749. Epub 2013 Aug 21. Nucleic Acids Res. 2013. PMID: 23965305 Free PMC article.
-
ATP-bound conformation of topoisomerase IV: a possible target for quinolones in Streptococcus pneumoniae.J Bacteriol. 2003 Oct;185(20):6137-46. doi: 10.1128/JB.185.20.6137-6146.2003. J Bacteriol. 2003. PMID: 14526026 Free PMC article.
-
What makes a type IIA topoisomerase a gyrase or a Topo IV?Nucleic Acids Res. 2021 Jun 21;49(11):6027-6042. doi: 10.1093/nar/gkab270. Nucleic Acids Res. 2021. PMID: 33905522 Free PMC article. Review.
-
Breakage-reunion domain of Streptococcus pneumoniae topoisomerase IV: crystal structure of a gram-positive quinolone target.PLoS One. 2007 Mar 21;2(3):e301. doi: 10.1371/journal.pone.0000301. PLoS One. 2007. PMID: 17375187 Free PMC article.
-
The mechanism of negative DNA supercoiling: a cascade of DNA-induced conformational changes prepares gyrase for strand passage.DNA Repair (Amst). 2014 Apr;16:23-34. doi: 10.1016/j.dnarep.2014.01.011. Epub 2014 Feb 22. DNA Repair (Amst). 2014. PMID: 24674625 Review.
Cited by
-
The pentapeptide-repeat protein, MfpA, interacts with mycobacterial DNA gyrase as a DNA T-segment mimic.Proc Natl Acad Sci U S A. 2021 Mar 16;118(11):e2016705118. doi: 10.1073/pnas.2016705118. Proc Natl Acad Sci U S A. 2021. PMID: 33836580 Free PMC article.
-
Structural basis for allosteric regulation of Human Topoisomerase IIα.Nat Commun. 2021 May 20;12(1):2962. doi: 10.1038/s41467-021-23136-6. Nat Commun. 2021. PMID: 34016969 Free PMC article.
-
A ubiquitin-like domain is required for stabilizing the N-terminal ATPase module of human SMCHD1.Commun Biol. 2019 Jul 10;2:255. doi: 10.1038/s42003-019-0499-y. eCollection 2019. Commun Biol. 2019. PMID: 31312724 Free PMC article.
-
DNA-Stimulated Liquid-Liquid phase separation by eukaryotic topoisomerase ii modulates catalytic function.Elife. 2022 Nov 7;11:e81786. doi: 10.7554/eLife.81786. Elife. 2022. PMID: 36342377 Free PMC article.
-
Structure-function analysis of the ATPase domain of African swine fever virus topoisomerase.mBio. 2024 Apr 10;15(4):e0308623. doi: 10.1128/mbio.03086-23. Epub 2024 Feb 27. mBio. 2024. PMID: 38411066 Free PMC article.
References
Publication types
MeSH terms
Substances
Grants and funding
LinkOut - more resources
Full Text Sources
Other Literature Sources
Miscellaneous