Imaging Myelination In Vivo Using Transparent Animal Models
- PMID: 29765846
- PMCID: PMC5928531
- DOI: 10.3233/BPL-160029
Imaging Myelination In Vivo Using Transparent Animal Models
Abstract
Myelination by oligodendrocytes in the central nervous system (CNS) and Schwann cells in the peripheral nervous system is essential for nervous system function and health. Despite its importance, we have a relatively poor understanding of the molecular and cellular mechanisms that regulate myelination in the living animal, particularly in the CNS. This is partly due to the fact that myelination commences around birth in mammals, by which time the CNS is complex and largely inaccessible, and thus very difficult to image live in its intact form. As a consequence, in recent years much effort has been invested in the use of smaller, simpler, transparent model organisms to investigate mechanisms of myelination in vivo. Although the majority of such studies have employed zebrafish, the Xenopus tadpole also represents an important complementary system with advantages for investigating myelin biology in vivo. Here we review how the natural features of zebrafish embryos and larvae and Xenopus tadpoles make them ideal systems for experimentally interrogating myelination by live imaging. We outline common transgenic technologies used to generate zebrafish and Xenopus that express fluorescent reporters, which can be used to image myelination. We also provide an extensive overview of the imaging modalities most commonly employed to date to image the nervous system in these transparent systems, and also emerging technologies that we anticipate will become widely used in studies of zebrafish and Xenopus myelination in the near future.
Keywords: Xenopus; Zebrafish; live-imaging; myelin; oligodendrocyte; schwann cell.
Figures
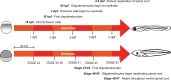

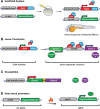
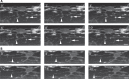
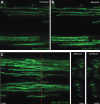
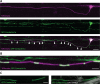
Similar articles
-
CNS Hypomyelination Disrupts Axonal Conduction and Behavior in Larval Zebrafish.J Neurosci. 2021 Nov 3;41(44):9099-9111. doi: 10.1523/JNEUROSCI.0842-21.2021. Epub 2021 Sep 20. J Neurosci. 2021. PMID: 34544838 Free PMC article.
-
Insights into mechanisms of central nervous system myelination using zebrafish.Glia. 2016 Mar;64(3):333-49. doi: 10.1002/glia.22897. Epub 2015 Aug 6. Glia. 2016. PMID: 26250418 Review.
-
Zebrafish as a model to investigate CNS myelination.Glia. 2015 Feb;63(2):177-93. doi: 10.1002/glia.22755. Epub 2014 Sep 27. Glia. 2015. PMID: 25263121 Free PMC article. Review.
-
Oligodendrocyte Development in the Absence of Their Target Axons In Vivo.PLoS One. 2016 Oct 7;11(10):e0164432. doi: 10.1371/journal.pone.0164432. eCollection 2016. PLoS One. 2016. PMID: 27716830 Free PMC article.
-
Visualization of myelination in GFP-transgenic zebrafish.Dev Dyn. 2010 Feb;239(2):592-7. doi: 10.1002/dvdy.22166. Dev Dyn. 2010. PMID: 19918882
Cited by
-
Clearing Your Mind: Mechanisms of Debris Clearance After Cell Death During Neural Development.Annu Rev Neurosci. 2022 Jul 8;45:177-198. doi: 10.1146/annurev-neuro-110920-022431. Epub 2022 Feb 28. Annu Rev Neurosci. 2022. PMID: 35226828 Free PMC article. Review.
-
Comparative Analysis of Neurotoxicity of Six Phthalates in Zebrafish Embryos.Toxics. 2021 Jan 7;9(1):5. doi: 10.3390/toxics9010005. Toxics. 2021. PMID: 33430197 Free PMC article.
-
In vivo imaging reveals mature Oligodendrocyte division in adult Zebrafish.Cell Regen. 2021 Jun 2;10(1):16. doi: 10.1186/s13619-021-00079-3. Cell Regen. 2021. PMID: 34075520 Free PMC article.
-
Myelin plasticity in adulthood and aging.Neurosci Lett. 2020 Jan 10;715:134645. doi: 10.1016/j.neulet.2019.134645. Epub 2019 Nov 22. Neurosci Lett. 2020. PMID: 31765728 Free PMC article. Review.
-
An automated high-resolution in vivo screen in zebrafish to identify chemical regulators of myelination.Elife. 2018 Jul 6;7:e35136. doi: 10.7554/eLife.35136. Elife. 2018. PMID: 29979149 Free PMC article.
References
Publication types
Grants and funding
LinkOut - more resources
Full Text Sources
Other Literature Sources
Research Materials