The substrate specificity of eukaryotic cytosolic chaperonin CCT
- PMID: 29735743
- PMCID: PMC5941184
- DOI: 10.1098/rstb.2017.0192
The substrate specificity of eukaryotic cytosolic chaperonin CCT
Abstract
The cytosolic chaperonin CCT (chaperonin containing TCP-1) is an ATP-dependent double-ring protein machine mediating the folding of members of the eukaryotic cytoskeletal protein families. The actins and tubulins are obligate substrates of CCT because they are completely dependent on CCT activity to reach their native states. Genetic and proteomic analysis of the CCT interactome in the yeast Saccharomyces cerevisiae revealed a CCT network of approximately 300 genes and proteins involved in many fundamental biological processes. We classified network members into sets such as substrates, CCT cofactors and CCT-mediated assembly processes. Many members of the 7-bladed propeller family of proteins are commonly found tightly bound to CCT isolated from human and plant cells and yeasts. The anaphase promoting complex (APC/C) cofactor propellers, Cdh1p and Cdc20p, are also obligate substrates since they both require CCT for folding and functional activation. In vitro translation analysis in prokaryotic and eukaryotic cell extracts of a set of yeast propellers demonstrates their highly differential interactions with CCT and GroEL (another chaperonin). Individual propeller proteins have idiosyncratic interaction modes with CCT because they emerged independently with neo-functions many times throughout eukaryotic evolution. We present a toy model in which cytoskeletal protein biogenesis and folding flux through CCT couples cell growth and size control to time dependent cell cycle mechanisms.This article is part of a discussion meeting issue 'Allostery and molecular machines'.
Keywords: 7-bladed WD40 propellers; APC/C; actin; chaperonin CCT; protein folding; tubulin.
© 2018 The Author(s).
Conflict of interest statement
We declare we have no competing interests.
Figures
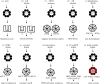
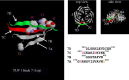
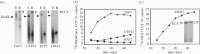
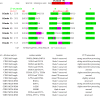
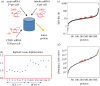
Similar articles
-
Quantitative actin folding reactions using yeast CCT purified via an internal tag in the CCT3/gamma subunit.J Mol Biol. 2006 Jul 7;360(2):484-96. doi: 10.1016/j.jmb.2006.05.003. Epub 2006 May 17. J Mol Biol. 2006. PMID: 16762366
-
The 'sequential allosteric ring' mechanism in the eukaryotic chaperonin-assisted folding of actin and tubulin.EMBO J. 2001 Aug 1;20(15):4065-75. doi: 10.1093/emboj/20.15.4065. EMBO J. 2001. PMID: 11483510 Free PMC article.
-
Yeast phosducin-like protein 2 acts as a stimulatory co-factor for the folding of actin by the chaperonin CCT via a ternary complex.J Mol Biol. 2009 Aug 7;391(1):192-206. doi: 10.1016/j.jmb.2009.06.003. Epub 2009 Jun 6. J Mol Biol. 2009. PMID: 19501098
-
Structure and function of a protein folding machine: the eukaryotic cytosolic chaperonin CCT.FEBS Lett. 2002 Oct 2;529(1):11-6. doi: 10.1016/s0014-5793(02)03180-0. FEBS Lett. 2002. PMID: 12354605 Review.
-
Mechanistic insights into protein folding by the eukaryotic chaperonin complex CCT.Biochem Soc Trans. 2022 Oct 31;50(5):1403-1414. doi: 10.1042/BST20220591. Biochem Soc Trans. 2022. PMID: 36196890 Free PMC article. Review.
Cited by
-
Chaperonin-Containing TCP1 Complex (CCT) Promotes Breast Cancer Growth Through Correlations With Key Cell Cycle Regulators.Front Oncol. 2021 Apr 30;11:663877. doi: 10.3389/fonc.2021.663877. eCollection 2021. Front Oncol. 2021. PMID: 33996588 Free PMC article.
-
Defining clinical subgroups and genotype-phenotype correlations in NBAS-associated disease across 110 patients.Genet Med. 2020 Mar;22(3):610-621. doi: 10.1038/s41436-019-0698-4. Epub 2019 Nov 25. Genet Med. 2020. PMID: 31761904
-
Allostery and molecular machines.Philos Trans R Soc Lond B Biol Sci. 2018 Jun 19;373(1749):20170173. doi: 10.1098/rstb.2017.0173. Philos Trans R Soc Lond B Biol Sci. 2018. PMID: 29735727 Free PMC article. No abstract available.
-
The role of molecular chaperone CCT/TRiC in translation elongation: A literature review.Heliyon. 2024 Apr 1;10(7):e29029. doi: 10.1016/j.heliyon.2024.e29029. eCollection 2024 Apr 15. Heliyon. 2024. PMID: 38596045 Free PMC article. Review.
-
Human Papillomavirus infection requires the CCT Chaperonin Complex.J Virol. 2021 May 10;95(11):e01943-20. doi: 10.1128/JVI.01943-20. Epub 2021 Mar 17. J Virol. 2021. PMID: 33731457 Free PMC article.
References
-
- Willison KR. 1999. Composition and function of the eukaryotic cytosolic chaperonin containing TCP-1. In Molecular chaperones and folding catalysts. Regulation, cellular function and mechanisms (ed. Bukau B.), pp. 555–571. Amsterdam, The Netherlands: Harwood Academic Publishers.
-
- Valpuesta JM, Carrascosa JL, Willison KR. 2005. Structure and function of the cytosolic chaperonin CCT. In Protein folding handbook (eds Buchner J, Kiefhaber T), pp. 725–755. Weinheim, Germany: Wiley-VCH Verlag GmbH.
Publication types
MeSH terms
Substances
LinkOut - more resources
Full Text Sources
Other Literature Sources
Molecular Biology Databases
Research Materials