The Many Roles of Ubiquitin in NF-κB Signaling
- PMID: 29642643
- PMCID: PMC6027159
- DOI: 10.3390/biomedicines6020043
The Many Roles of Ubiquitin in NF-κB Signaling
Abstract
The nuclear factor κB (NF-κB) signaling pathway ubiquitously controls cell growth and survival in basic conditions as well as rapid resetting of cellular functions following environment changes or pathogenic insults. Moreover, its deregulation is frequently observed during cell transformation, chronic inflammation or autoimmunity. Understanding how it is properly regulated therefore is a prerequisite to managing these adverse situations. Over the last years evidence has accumulated showing that ubiquitination is a key process in NF-κB activation and its resolution. Here, we examine the various functions of ubiquitin in NF-κB signaling and more specifically, how it controls signal transduction at the molecular level and impacts in vivo on NF-κB regulated cellular processes.
Keywords: nuclear factor κB; signal transduction; ubiquitin; ubiquitination/deubiquitination.
Conflict of interest statement
The authors declare no conflict of interest.
Figures
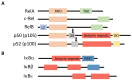
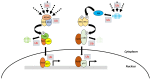
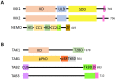
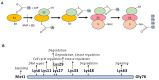
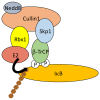
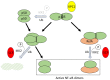
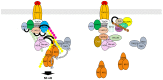
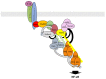
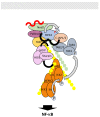
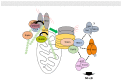
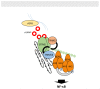
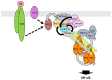
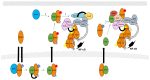
Similar articles
-
TRAF Family Member-associated NF-κB Activator (TANK) Inhibits Genotoxic Nuclear Factor κB Activation by Facilitating Deubiquitinase USP10-dependent Deubiquitination of TRAF6 Ligase.J Biol Chem. 2015 May 22;290(21):13372-85. doi: 10.1074/jbc.M115.643767. Epub 2015 Apr 10. J Biol Chem. 2015. PMID: 25861989 Free PMC article.
-
The role of ubiquitin in NF-kappaB regulatory pathways.Annu Rev Biochem. 2009;78:769-96. doi: 10.1146/annurev.biochem.78.070907.102750. Annu Rev Biochem. 2009. PMID: 19489733 Review.
-
Diverse ubiquitin signaling in NF-κB activation.Trends Cell Biol. 2012 Jul;22(7):355-64. doi: 10.1016/j.tcb.2012.04.001. Epub 2012 Apr 28. Trends Cell Biol. 2012. PMID: 22543051 Review.
-
Low nuclear body formation and tax SUMOylation do not prevent NF-kappaB promoter activation.Retrovirology. 2012 Sep 25;9:77. doi: 10.1186/1742-4690-9-77. Retrovirology. 2012. PMID: 23009398 Free PMC article.
-
Linear ubiquitination-mediated NF-κB regulation and its related disorders.J Biochem. 2013 Oct;154(4):313-23. doi: 10.1093/jb/mvt079. Epub 2013 Aug 21. J Biochem. 2013. PMID: 23969028 Review.
Cited by
-
Echinococcus granulosus Protoscoleces-Derived Exosome-like Vesicles and Egr-miR-277a-3p Promote Dendritic Cell Maturation and Differentiation.Cells. 2022 Oct 14;11(20):3220. doi: 10.3390/cells11203220. Cells. 2022. PMID: 36291088 Free PMC article.
-
The Nuclear Factor Kappa B (NF-kB) signaling in cancer development and immune diseases.Genes Dis. 2020 Jul 18;8(3):287-297. doi: 10.1016/j.gendis.2020.06.005. eCollection 2021 May. Genes Dis. 2020. PMID: 33997176 Free PMC article. Review.
-
MicroRNA-941 regulates the proliferation of breast cancer cells by altering histone H3 Ser 10 phosphorylation.Sci Rep. 2020 Oct 21;10(1):17954. doi: 10.1038/s41598-020-74847-7. Sci Rep. 2020. PMID: 33087811 Free PMC article.
-
Ubiquitination and deubiquitination in cancer: from mechanisms to novel therapeutic approaches.Mol Cancer. 2024 Jul 25;23(1):148. doi: 10.1186/s12943-024-02046-3. Mol Cancer. 2024. PMID: 39048965 Free PMC article. Review.
-
DUBs Activating the Hedgehog Signaling Pathway: A Promising Therapeutic Target in Cancer.Cancers (Basel). 2020 Jun 10;12(6):1518. doi: 10.3390/cancers12061518. Cancers (Basel). 2020. PMID: 32531973 Free PMC article. Review.
References
-
- Aggarwal B.B., Takada Y., Shishodia S., Gutierrez A.M., Oommen O.V., Ichikawa H., Baba Y., Kumar A. Nuclear transcription factor NF-κB: Role in biology and medicine. Indian J. Exp. Biol. 2004;42:341–353. - PubMed
Publication types
LinkOut - more resources
Full Text Sources
Other Literature Sources