lncRNA Epigenetic Landscape Analysis Identifies EPIC1 as an Oncogenic lncRNA that Interacts with MYC and Promotes Cell-Cycle Progression in Cancer
- PMID: 29622465
- PMCID: PMC6143179
- DOI: 10.1016/j.ccell.2018.03.006
lncRNA Epigenetic Landscape Analysis Identifies EPIC1 as an Oncogenic lncRNA that Interacts with MYC and Promotes Cell-Cycle Progression in Cancer
Abstract
We characterized the epigenetic landscape of genes encoding long noncoding RNAs (lncRNAs) across 6,475 tumors and 455 cancer cell lines. In stark contrast to the CpG island hypermethylation phenotype in cancer, we observed a recurrent hypomethylation of 1,006 lncRNA genes in cancer, including EPIC1 (epigenetically-induced lncRNA1). Overexpression of EPIC1 is associated with poor prognosis in luminal B breast cancer patients and enhances tumor growth in vitro and in vivo. Mechanistically, EPIC1 promotes cell-cycle progression by interacting with MYC through EPIC1's 129-283 nt region. EPIC1 knockdown reduces the occupancy of MYC to its target genes (e.g., CDKN1A, CCNA2, CDC20, and CDC45). MYC depletion abolishes EPIC1's regulation of MYC target and luminal breast cancer tumorigenesis in vitro and in vivo.
Keywords: CIMP; ENSG00000224271; EPIC1; LOC284930; MYC; P21; TCGA pan-cancer; breast cancer; long noncoding RNA.
Copyright © 2018 Elsevier Inc. All rights reserved.
Figures
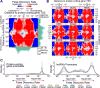
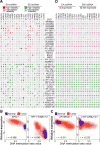
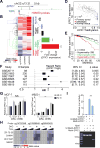
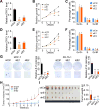
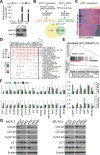
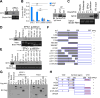
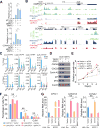
Similar articles
-
MYC-binding lncRNA EPIC1 promotes AKT-mTORC1 signaling and rapamycin resistance in breast and ovarian cancer.Mol Carcinog. 2020 Oct;59(10):1188-1198. doi: 10.1002/mc.23248. Epub 2020 Aug 18. Mol Carcinog. 2020. PMID: 32810332 Free PMC article.
-
Long non-coding RNA EPIC1 promotes cholangiocarcinoma cell growth.Biochem Biophys Res Commun. 2018 Oct 12;504(4):654-659. doi: 10.1016/j.bbrc.2018.08.174. Epub 2018 Sep 8. Biochem Biophys Res Commun. 2018. PMID: 30205958
-
Long non-coding RNA EPIC1 promotes human lung cancer cell growth.Biochem Biophys Res Commun. 2018 Sep 10;503(3):1342-1348. doi: 10.1016/j.bbrc.2018.07.046. Epub 2018 Jul 17. Biochem Biophys Res Commun. 2018. PMID: 30029875
-
Long Noncoding RNA GAS5 in Breast Cancer: Epigenetic Mechanisms and Biological Functions.Int J Mol Sci. 2021 Jun 24;22(13):6810. doi: 10.3390/ijms22136810. Int J Mol Sci. 2021. PMID: 34202777 Free PMC article. Review.
-
Crosstalk between oncogenic MYC and noncoding RNAs in cancer.Semin Cancer Biol. 2021 Oct;75:62-71. doi: 10.1016/j.semcancer.2020.10.014. Epub 2020 Nov 4. Semin Cancer Biol. 2021. PMID: 33160022 Review.
Cited by
-
Construction and Validation of Novel Diagnostic and Prognostic DNA Methylation Signatures for Hepatocellular Carcinoma.Front Genet. 2020 Aug 13;11:906. doi: 10.3389/fgene.2020.00906. eCollection 2020. Front Genet. 2020. PMID: 32922438 Free PMC article.
-
Beyond the Genome: Deciphering the Role of MALAT1 in Breast Cancer Progression.Curr Genomics. 2024;25(5):343-357. doi: 10.2174/0113892029305656240503045154. Epub 2024 May 22. Curr Genomics. 2024. PMID: 39323624 Free PMC article. Review.
-
Non-coding RNAs as therapeutic targets in cancer and its clinical application.J Pharm Anal. 2024 Jul;14(7):100947. doi: 10.1016/j.jpha.2024.02.001. Epub 2024 Feb 8. J Pharm Anal. 2024. PMID: 39149142 Free PMC article. Review.
-
An RNA-sequencing-based transcriptome for a significantly prognostic novel driver signature identification in bladder urothelial carcinoma.PeerJ. 2020 Jul 21;8:e9422. doi: 10.7717/peerj.9422. eCollection 2020. PeerJ. 2020. PMID: 32742772 Free PMC article.
-
LncRNA LUCAT1 as a novel prognostic biomarker for patients with papillary thyroid cancer.Sci Rep. 2019 Oct 7;9(1):14374. doi: 10.1038/s41598-019-50913-7. Sci Rep. 2019. PMID: 31591432 Free PMC article.
References
-
- Amati B, Brooks MW, Levy N, Littlewood TD, Evan GI, Land H. Oncogenic activity of the c-Myc protein requires dimerization with Max. Cell. 1993;72:233–245. - PubMed
Publication types
MeSH terms
Substances
Grants and funding
LinkOut - more resources
Full Text Sources
Other Literature Sources
Medical
Research Materials
Miscellaneous