Proline hydroxylation in collagen supports integrin binding by two distinct mechanisms
- PMID: 29615493
- PMCID: PMC5961056
- DOI: 10.1074/jbc.RA118.002200
Proline hydroxylation in collagen supports integrin binding by two distinct mechanisms
Abstract
Collagens are the most abundant extracellular matrix proteins in vertebrates and have a characteristic triple-helix structure. Hydroxylation of proline residues is critical for helix stability, and diminished prolyl hydroxylase activity causes wide-spread defects in connective tissues. Still, the role of proline hydroxylation in the binding of collagen receptors such as integrins is unclear. Here, we isolated skin collagen from genetically modified mice having reduced prolyl 4-hydroxylase activity. At room temperature, the reduced proline hydroxylation did not affect interactions with the recombinant integrin α2I domain, but at 37 °C, collagen hydroxylation correlated with the avidity of α2I domain binding. Of note, LC-MS/MS analysis of isolated skin collagens revealed no major changes in the hydroxyproline content of the main integrin-binding sites. Thus, the disrupted α2I domain binding at physiological temperatures was most likely due to structural destabilization of the collagenous helix. Integrin α2I binding to the triple-helical GFPGER motif was slightly weaker than to GFOGER (O = hydroxyproline). This phenomenon was more prominent when α1 integrin was tested. Integrin α1β1 expressed on CHO cells and recombinant α1I domain showed remarkably slower binding velocity and weaker avidity to GFPGER when compared with GFOGER. Structural modeling revealed the critical interaction between Arg-218 in α1I and the hydroxyproline residue in the integrin-binding motif. The role of Arg-218 was further validated by testing a variant R218D α1I domain in solid-phase binding assays. Thus, our results show that the lack of proline hydroxylation in collagen can affect integrin binding by a direct mechanism and via structural destabilization of the triple helix.
Keywords: cell adhesion; collagen; connective tissue; hydroxyproline; integrin; post-translational modification (PTM).
© 2018 Sipilä et al.
Conflict of interest statement
J. M. owns equity in FibroGen Inc., which develops hypoxia-inducible factor P4H inhibitors as potential therapeutics. This company supports hypoxia response research in the J. M. group
Figures
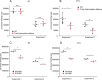
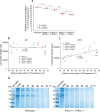
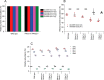
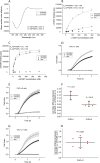
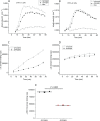
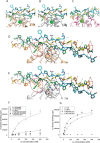
Similar articles
-
Role of prolyl hydroxylation in the molecular interactions of collagens.Essays Biochem. 2019 Sep 13;63(3):325-335. doi: 10.1042/EBC20180053. Print 2019 Sep 13. Essays Biochem. 2019. PMID: 31350381 Free PMC article. Review.
-
Prolyl hydroxylation of collagen type I is required for efficient binding to integrin alpha 1 beta 1 and platelet glycoprotein VI but not to alpha 2 beta 1.J Biol Chem. 2003 Aug 8;278(32):29873-9. doi: 10.1074/jbc.M304073200. Epub 2003 May 27. J Biol Chem. 2003. PMID: 12771137
-
The fibril-associated collagen IX provides a novel mechanism for cell adhesion to cartilaginous matrix.J Biol Chem. 2004 Dec 3;279(49):51677-87. doi: 10.1074/jbc.M409412200. Epub 2004 Sep 21. J Biol Chem. 2004. PMID: 15383545
-
Hydroxyproline Ring Pucker Causes Frustration of Helix Parameters in the Collagen Triple Helix.Sci Rep. 2015 Jul 29;5:12556. doi: 10.1038/srep12556. Sci Rep. 2015. PMID: 26220399 Free PMC article.
-
The importance of proline residues in the structure, stability and susceptibility to proteolytic degradation of collagens.Amino Acids. 2008 Nov;35(4):703-10. doi: 10.1007/s00726-008-0073-2. Epub 2008 Apr 23. Amino Acids. 2008. PMID: 18431533 Review.
Cited by
-
Selection of different endothelialization modes and different seed cells for tissue-engineered vascular graft.Bioact Mater. 2021 Feb 6;6(8):2557-2568. doi: 10.1016/j.bioactmat.2020.12.021. eCollection 2021 Aug. Bioact Mater. 2021. PMID: 33665496 Free PMC article. Review.
-
Collagen fiber regulation in human pediatric aortic valve development and disease.Sci Rep. 2021 May 7;11(1):9751. doi: 10.1038/s41598-021-89164-w. Sci Rep. 2021. PMID: 33963260 Free PMC article.
-
Zonal regulation of collagen-type proteins and posttranslational modifications in prostatic benign and cancer tissues by imaging mass spectrometry.Prostate. 2020 Sep;80(13):1071-1086. doi: 10.1002/pros.24031. Epub 2020 Jul 20. Prostate. 2020. PMID: 32687633 Free PMC article.
-
Proteomic Analysis of Decellularized Extracellular Matrix: Achieving a Competent Biomaterial for Osteogenesis.Biomed Res Int. 2022 Oct 11;2022:6884370. doi: 10.1155/2022/6884370. eCollection 2022. Biomed Res Int. 2022. PMID: 36267842 Free PMC article.
-
The Spatial Extracellular Proteomic Tumor Microenvironment Distinguishes Molecular Subtypes of Hepatocellular Carcinoma.J Proteome Res. 2024 Sep 6;23(9):3791-3805. doi: 10.1021/acs.jproteome.4c00099. Epub 2024 Jul 9. J Proteome Res. 2024. PMID: 38980715 Free PMC article.
References
-
- Kivirikko K. I., Myllylä R., and Pihlajaniemi T. (1992) Prolyl hydroxylase, protein disulfide isomerase, and other structurally related proteins. in Post-Translational Modifications of Proteins (Harding J. J., and Crabbe M. J. C., eds) pp. 1–51, CRC Press, Boca Raton, FL
-
- Helaakoski T., Annunen P., Vuori K., MacNeil I. A., Pihlajaniemi T., and Kivirikko K. I. (1995) Cloning, baculovirus expression, and characterization of a second mouse prolyl 4-hydroxylase α-subunit isoform: formation of an α2β2 tetramer with the protein disulfide-isomerase/β subunit. Proc. Natl. Acad. Sci. U.S.A. 92, 4427–4431 10.1073/pnas.92.10.4427 - DOI - PMC - PubMed
Publication types
MeSH terms
Substances
Associated data
- Actions
- Actions
LinkOut - more resources
Full Text Sources
Other Literature Sources
Molecular Biology Databases