NAA80 is actin's N-terminal acetyltransferase and regulates cytoskeleton assembly and cell motility
- PMID: 29581253
- PMCID: PMC5924898
- DOI: 10.1073/pnas.1718336115
NAA80 is actin's N-terminal acetyltransferase and regulates cytoskeleton assembly and cell motility
Abstract
Actin, one of the most abundant proteins in nature, participates in countless cellular functions ranging from organelle trafficking and pathogen motility to cell migration and regulation of gene transcription. Actin's cellular activities depend on the dynamic transition between its monomeric and filamentous forms, a process exquisitely regulated in cells by a large number of actin-binding and signaling proteins. Additionally, several posttranslational modifications control the cellular functions of actin, including most notably N-terminal (Nt)-acetylation, a prevalent modification throughout the animal kingdom. However, the biological role and mechanism of actin Nt-acetylation are poorly understood, and the identity of actin's N-terminal acetyltransferase (NAT) has remained a mystery. Here, we reveal that NAA80, a suggested NAT enzyme whose substrate specificity had not been characterized, is Nt-acetylating actin. We further show that actin Nt-acetylation plays crucial roles in cytoskeletal assembly in vitro and in cells. The absence of Nt-acetylation leads to significant differences in the rates of actin filament depolymerization and elongation, including elongation driven by formins, whereas filament nucleation by the Arp2/3 complex is mostly unaffected. NAA80-knockout cells display severely altered cytoskeletal organization, including an increase in the ratio of filamentous to globular actin, increased filopodia and lamellipodia formation, and accelerated cell motility. Together, the results demonstrate NAA80's role as actin's NAT and reveal a crucial role for actin Nt-acetylation in the control of cytoskeleton structure and dynamics.
Keywords: N-terminal acetylation; NAA80; NAT; actin; cell motility.
Copyright © 2018 the Author(s). Published by PNAS.
Conflict of interest statement
The authors declare no conflict of interest.
Figures
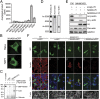
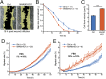
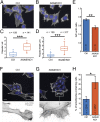
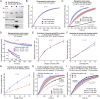
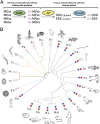
Comment in
-
NATure of actin amino-terminal acetylation.Proc Natl Acad Sci U S A. 2018 Apr 24;115(17):4314-4316. doi: 10.1073/pnas.1803804115. Epub 2018 Apr 9. Proc Natl Acad Sci U S A. 2018. PMID: 29632202 Free PMC article. No abstract available.
Similar articles
-
Actin's N-terminal acetyltransferase uncovered.Cytoskeleton (Hoboken). 2018 Jul;75(7):318-322. doi: 10.1002/cm.21455. Epub 2018 Aug 26. Cytoskeleton (Hoboken). 2018. PMID: 30084538 Free PMC article.
-
N-terminal acetylation of actin by NAA80 is essential for structural integrity of the Golgi apparatus.Exp Cell Res. 2020 May 15;390(2):111961. doi: 10.1016/j.yexcr.2020.111961. Epub 2020 Mar 21. Exp Cell Res. 2020. PMID: 32209306 Free PMC article.
-
Structural determinants and cellular environment define processed actin as the sole substrate of the N-terminal acetyltransferase NAA80.Proc Natl Acad Sci U S A. 2018 Apr 24;115(17):4405-4410. doi: 10.1073/pnas.1719251115. Epub 2018 Mar 26. Proc Natl Acad Sci U S A. 2018. PMID: 29581307 Free PMC article.
-
Co-translational, Post-translational, and Non-catalytic Roles of N-Terminal Acetyltransferases.Mol Cell. 2019 Mar 21;73(6):1097-1114. doi: 10.1016/j.molcel.2019.02.007. Epub 2019 Mar 13. Mol Cell. 2019. PMID: 30878283 Free PMC article. Review.
-
NATs at a glance.J Cell Sci. 2023 Jul 15;136(14):jcs260766. doi: 10.1242/jcs.260766. Epub 2023 Jul 18. J Cell Sci. 2023. PMID: 37462250 Review.
Cited by
-
Dynamics-function relationship in the catalytic domains of N-terminal acetyltransferases.Comput Struct Biotechnol J. 2020 Mar 3;18:532-547. doi: 10.1016/j.csbj.2020.02.017. eCollection 2020. Comput Struct Biotechnol J. 2020. PMID: 32206212 Free PMC article.
-
Optimized bisubstrate inhibitors for the actin N-terminal acetyltransferase NAA80.Front Chem. 2023 Jun 20;11:1202501. doi: 10.3389/fchem.2023.1202501. eCollection 2023. Front Chem. 2023. PMID: 37408560 Free PMC article.
-
Overlap of NatA and IAP substrates implicates N-terminal acetylation in protein stabilization.Sci Adv. 2021 Jan 15;7(3):eabc8590. doi: 10.1126/sciadv.abc8590. Print 2021 Jan. Sci Adv. 2021. PMID: 33523899 Free PMC article.
-
N-Terminal Acetylation Stabilizes SIGMA FACTOR BINDING PROTEIN1 Involved in Salicylic Acid-Primed Cell Death.Plant Physiol. 2020 May;183(1):358-370. doi: 10.1104/pp.19.01417. Epub 2020 Mar 5. Plant Physiol. 2020. PMID: 32139475 Free PMC article.
-
lncRNA ADAMTS9-AS1/circFN1 Competitively Binds to miR-206 to Elevate the Expression of ACTB, Thus Inducing Hypertrophic Cardiomyopathy.Oxid Med Cell Longev. 2022 Mar 31;2022:1450610. doi: 10.1155/2022/1450610. eCollection 2022. Oxid Med Cell Longev. 2022. PMID: 35401927 Free PMC article.
References
-
- Sheterline P, Clayton J, Sparrow J. Actin. Protein Profile. 1995;2:1–103. - PubMed
Publication types
MeSH terms
Substances
Grants and funding
LinkOut - more resources
Full Text Sources
Other Literature Sources
Molecular Biology Databases
Research Materials