PARP-1 inhibition with or without ionizing radiation confers reactive oxygen species-mediated cytotoxicity preferentially to cancer cells with mutant TP53
- PMID: 29511347
- PMCID: PMC5970015
- DOI: 10.1038/s41388-018-0130-6
PARP-1 inhibition with or without ionizing radiation confers reactive oxygen species-mediated cytotoxicity preferentially to cancer cells with mutant TP53
Abstract
Biomarkers and mechanisms of poly (ADP-ribose) polymerase (PARP) inhibitor-mediated cytotoxicity in tumor cells lacking a BRCA-mutant or BRCA-like phenotype are poorly defined. We sought to explore the utility of PARP-1 inhibitor (PARPi) treatment with/without ionizing radiation in muscle-invasive bladder cancer (MIBC), which has poor therapeutic outcomes. We assessed the DNA damaging and cytotoxic effects of the PARPi olaparib in nine bladder cancer cell lines. Olaparib radiosensitized all cell lines with dose enhancement factors from 1.22 to 2.27. Radiosensitization was correlated with the induction of potentially lethal DNA double-strand breaks (DSB) but not with RAD51 foci formation. The ability of olaparib to radiosensitize MIBC cells was linked to the extent of cell kill achieved with the drug alone. Unexpectedly, increased levels of reactive oxygen species (ROS) resulting from PARPi treatment were the cause of DSB throughout the cell cycle in vitro and in vivo. ROS originated from mitochondria and were required for the radiosensitizing effects of olaparib. Consistent with the role of TP53 in ROS regulation, loss of p53 function enhanced radiosensitization by olaparib in non-isogenic and isogenic cell line models and was associated with increased PARP-1 expression in bladder cancer cell lines and tumors. Impairment of ATM in addition to p53 loss resulted in an even more pronounced radiosensitization. In conclusion, ROS suppression by PARP-1 in MIBC is a potential therapeutic target either for PARPi combined with radiation or drug alone treatment. The TP53 and ATM genes, commonly mutated in MIBC and other cancers, are candidate biomarkers of PARPi-mediated radiosensitization.
Conflict of interest statement
The authors disclose no potential conflicts of interest.
Figures
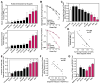
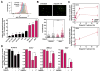
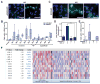
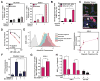
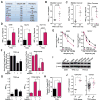
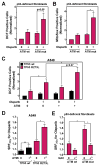
Similar articles
-
The combination of olaparib and camptothecin for effective radiosensitization.Radiat Oncol. 2012 Apr 23;7:62. doi: 10.1186/1748-717X-7-62. Radiat Oncol. 2012. PMID: 22524618 Free PMC article.
-
PARP1 Trapping and DNA Replication Stress Enhance Radiosensitization with Combined WEE1 and PARP Inhibitors.Mol Cancer Res. 2018 Feb;16(2):222-232. doi: 10.1158/1541-7786.MCR-17-0455. Epub 2017 Nov 13. Mol Cancer Res. 2018. PMID: 29133592 Free PMC article.
-
Poly (ADP-ribose) polymerase inhibitors sensitize cancer cells to hypofractionated radiotherapy through altered selection of DNA double-strand break repair pathways.Int J Radiat Biol. 2022;98(7):1222-1234. doi: 10.1080/09553002.2022.2020357. Epub 2022 Jan 4. Int J Radiat Biol. 2022. PMID: 34919022
-
Talazoparib enhances resection at DSBs and renders HR-proficient cancer cells susceptible to Polθ inhibition.Radiother Oncol. 2024 Nov;200:110475. doi: 10.1016/j.radonc.2024.110475. Epub 2024 Aug 13. Radiother Oncol. 2024. PMID: 39147034
-
Detection of a novel, primate-specific 'kill switch' tumor suppression mechanism that may fundamentally control cancer risk in humans: an unexpected twist in the basic biology of TP53.Endocr Relat Cancer. 2018 Nov;25(11):R497-R517. doi: 10.1530/ERC-18-0241. Epub 2018 Jun 25. Endocr Relat Cancer. 2018. PMID: 29941676 Free PMC article. Review.
Cited by
-
Misrepair in Context: TGFβ Regulation of DNA Repair.Front Oncol. 2019 Aug 27;9:799. doi: 10.3389/fonc.2019.00799. eCollection 2019. Front Oncol. 2019. PMID: 31552165 Free PMC article. Review.
-
PARP inhibition promotes ferroptosis via repressing SLC7A11 and synergizes with ferroptosis inducers in BRCA-proficient ovarian cancer.Redox Biol. 2021 Jun;42:101928. doi: 10.1016/j.redox.2021.101928. Epub 2021 Mar 5. Redox Biol. 2021. PMID: 33722571 Free PMC article.
-
The synthetic lethality of targeting cell cycle checkpoints and PARPs in cancer treatment.J Hematol Oncol. 2022 Oct 17;15(1):147. doi: 10.1186/s13045-022-01360-x. J Hematol Oncol. 2022. PMID: 36253861 Free PMC article. Review.
-
CCDC6 and USP7 expression levels suggest novel treatment options in high-grade urothelial bladder cancer.J Exp Clin Cancer Res. 2019 Feb 20;38(1):90. doi: 10.1186/s13046-019-1087-1. J Exp Clin Cancer Res. 2019. PMID: 30786932 Free PMC article.
-
Nuclear translocation of the receptor tyrosine kinase c-MET reduces the treatment efficacies of olaparib and gemcitabine in pancreatic ductal adenocarcinoma cells.Am J Cancer Res. 2021 Jan 1;11(1):236-250. eCollection 2021. Am J Cancer Res. 2021. PMID: 33520371 Free PMC article.
References
-
- Ameziane-El-Hassani R, Dupuy C. Detection of Intracellular Reactive Oxygen Species (CM-H2DCFDA) Bio-protocol. 2013;3:e313.
-
- Audebert M, Salles B, Calsou P. Involvement of poly(ADP-ribose) polymerase-1 and XRCC1/DNA ligase III in an alternative route for DNA double-strand breaks rejoining. J Biol Chem. 2004;279:55117–55126. - PubMed
Publication types
MeSH terms
Substances
Grants and funding
LinkOut - more resources
Full Text Sources
Other Literature Sources
Medical
Research Materials
Miscellaneous