A facile approach to enhance antigen response for personalized cancer vaccination
- PMID: 29507416
- PMCID: PMC5970019
- DOI: 10.1038/s41563-018-0028-2
A facile approach to enhance antigen response for personalized cancer vaccination
Abstract
Existing strategies to enhance peptide immunogenicity for cancer vaccination generally require direct peptide alteration, which, beyond practical issues, may impact peptide presentation and result in vaccine variability. Here, we report a simple adsorption approach using polyethyleneimine (PEI) in a mesoporous silica microrod (MSR) vaccine to enhance antigen immunogenicity. The MSR-PEI vaccine significantly enhanced host dendritic cell activation and T-cell response over the existing MSR vaccine and bolus vaccine formulations. Impressively, a single injection of the MSR-PEI vaccine using an E7 peptide completely eradicated large, established TC-1 tumours in about 80% of mice and generated immunological memory. When immunized with a pool of B16F10 or CT26 neoantigens, the MSR-PEI vaccine eradicated established lung metastases, controlled tumour growth and synergized with anti-CTLA4 therapy. Our findings from three independent tumour models suggest that the MSR-PEI vaccine approach may serve as a facile and powerful multi-antigen platform to enable robust personalized cancer vaccination.
Conflict of interest statement
The authors declare no competing financial interests.
Figures
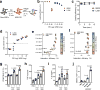
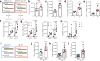
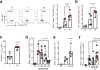
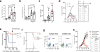
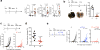
Comment in
-
Smart delivery of vaccines.Nat Mater. 2018 Jun;17(6):482-483. doi: 10.1038/s41563-018-0085-6. Nat Mater. 2018. PMID: 29795220 No abstract available.
Similar articles
-
Designer vaccine nanodiscs for personalized cancer immunotherapy.Nat Mater. 2017 Apr;16(4):489-496. doi: 10.1038/nmat4822. Epub 2016 Dec 26. Nat Mater. 2017. PMID: 28024156 Free PMC article.
-
GTL001 and bivalent CyaA-based therapeutic vaccine strategies against human papillomavirus and other tumor-associated antigens induce effector and memory T-cell responses that inhibit tumor growth.Vaccine. 2017 Mar 13;35(11):1509-1516. doi: 10.1016/j.vaccine.2017.01.074. Epub 2017 Feb 10. Vaccine. 2017. PMID: 28196735
-
Covalent Conjugation of Peptide Antigen to Mesoporous Silica Rods to Enhance Cellular Responses.Bioconjug Chem. 2018 Mar 21;29(3):733-741. doi: 10.1021/acs.bioconjchem.7b00656. Epub 2018 Jan 10. Bioconjug Chem. 2018. PMID: 29318872
-
The present status and future prospects of peptide-based cancer vaccines.Int Immunol. 2016 Jul;28(7):319-28. doi: 10.1093/intimm/dxw027. Epub 2016 May 28. Int Immunol. 2016. PMID: 27235694 Review.
-
Therapeutic vaccination with tumor cells that engage CD137.J Mol Med (Berl). 2003 Feb;81(2):71-86. doi: 10.1007/s00109-002-0413-8. Epub 2003 Feb 8. J Mol Med (Berl). 2003. PMID: 12601523 Review.
Cited by
-
Efficient Lymph Node-Targeted Delivery of Personalized Cancer Vaccines with Reactive Oxygen Species-Inducing Reduced Graphene Oxide Nanosheets.ACS Nano. 2020 Oct 27;14(10):13268-13278. doi: 10.1021/acsnano.0c05062. Epub 2020 Sep 15. ACS Nano. 2020. PMID: 32902245 Free PMC article.
-
Biomaterials for vaccine-based cancer immunotherapy.J Control Release. 2018 Dec 28;292:256-276. doi: 10.1016/j.jconrel.2018.10.008. Epub 2018 Oct 9. J Control Release. 2018. PMID: 30312721 Free PMC article. Review.
-
Intratumourally injected alum-tethered cytokines elicit potent and safer local and systemic anticancer immunity.Nat Biomed Eng. 2022 Feb;6(2):129-143. doi: 10.1038/s41551-021-00831-9. Epub 2022 Jan 10. Nat Biomed Eng. 2022. PMID: 35013574 Free PMC article.
-
Development and validation of a clinical prediction model to estimate the risk of critical patients with COVID-19.J Med Virol. 2022 Mar;94(3):1104-1114. doi: 10.1002/jmv.27428. Epub 2021 Nov 6. J Med Virol. 2022. PMID: 34716705 Free PMC article.
-
Robust Antigen-Specific T Cell Activation within Injectable 3D Synthetic Nanovaccine Depots.ACS Biomater Sci Eng. 2021 Dec 13;7(12):5622-5632. doi: 10.1021/acsbiomaterials.0c01648. Epub 2021 Nov 4. ACS Biomater Sci Eng. 2021. PMID: 34734689 Free PMC article.
References
Publication types
MeSH terms
Substances
Grants and funding
LinkOut - more resources
Full Text Sources
Other Literature Sources
Medical