TADs are 3D structural units of higher-order chromosome organization in Drosophila
- PMID: 29503869
- PMCID: PMC5829972
- DOI: 10.1126/sciadv.aar8082
TADs are 3D structural units of higher-order chromosome organization in Drosophila
Abstract
Deciphering the rules of genome folding in the cell nucleus is essential to understand its functions. Recent chromosome conformation capture (Hi-C) studies have revealed that the genome is partitioned into topologically associating domains (TADs), which demarcate functional epigenetic domains defined by combinations of specific chromatin marks. However, whether TADs are true physical units in each cell nucleus or whether they reflect statistical frequencies of measured interactions within cell populations is unclear. Using a combination of Hi-C, three-dimensional (3D) fluorescent in situ hybridization, super-resolution microscopy, and polymer modeling, we provide an integrative view of chromatin folding in Drosophila. We observed that repressed TADs form a succession of discrete nanocompartments, interspersed by less condensed active regions. Single-cell analysis revealed a consistent TAD-based physical compartmentalization of the chromatin fiber, with some degree of heterogeneity in intra-TAD conformations and in cis and trans inter-TAD contact events. These results indicate that TADs are fundamental 3D genome units that engage in dynamic higher-order inter-TAD connections. This domain-based architecture is likely to play a major role in regulatory transactions during DNA-dependent processes.
Figures
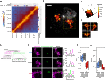
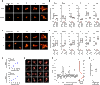
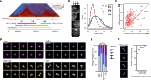
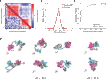
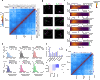
Similar articles
-
Active chromatin and transcription play a key role in chromosome partitioning into topologically associating domains.Genome Res. 2016 Jan;26(1):70-84. doi: 10.1101/gr.196006.115. Epub 2015 Oct 30. Genome Res. 2016. PMID: 26518482 Free PMC article.
-
Unraveling the mechanisms of chromatin fibril packaging.Nucleus. 2016 May 3;7(3):319-24. doi: 10.1080/19491034.2016.1190896. Nucleus. 2016. PMID: 27249516 Free PMC article.
-
Order and stochasticity in the folding of individual Drosophila genomes.Nat Commun. 2021 Jan 4;12(1):41. doi: 10.1038/s41467-020-20292-z. Nat Commun. 2021. PMID: 33397980 Free PMC article.
-
Multi-Scale Organization of the Drosophila melanogaster Genome.Genes (Basel). 2021 May 27;12(6):817. doi: 10.3390/genes12060817. Genes (Basel). 2021. PMID: 34071789 Free PMC article. Review.
-
Making Sense of the Tangle: Insights into Chromatin Folding and Gene Regulation.Genes (Basel). 2016 Sep 23;7(10):71. doi: 10.3390/genes7100071. Genes (Basel). 2016. PMID: 27669308 Free PMC article. Review.
Cited by
-
The Wg and Dpp morphogens regulate gene expression by modulating the frequency of transcriptional bursts.Elife. 2020 Jun 22;9:e56076. doi: 10.7554/eLife.56076. Elife. 2020. PMID: 32568073 Free PMC article.
-
Topological screen identifies hundreds of Cp190- and CTCF-dependent Drosophila chromatin insulator elements.Sci Adv. 2023 Feb 3;9(5):eade0090. doi: 10.1126/sciadv.ade0090. Epub 2023 Feb 3. Sci Adv. 2023. PMID: 36735780 Free PMC article.
-
RASER-FISH: non-denaturing fluorescence in situ hybridization for preservation of three-dimensional interphase chromatin structure.Nat Protoc. 2022 May;17(5):1306-1331. doi: 10.1038/s41596-022-00685-8. Epub 2022 Apr 4. Nat Protoc. 2022. PMID: 35379945 Review.
-
Enhancer-gene specificity in development and disease.Development. 2022 Jun 1;149(11):dev186536. doi: 10.1242/dev.186536. Epub 2022 Jun 10. Development. 2022. PMID: 35686641 Free PMC article. Review.
-
Chromatin Organization and Function in Drosophila.Cells. 2021 Sep 8;10(9):2362. doi: 10.3390/cells10092362. Cells. 2021. PMID: 34572010 Free PMC article. Review.
References
-
- Bonev B., Cavalli G., Organization and function of the 3D genome. Nat. Rev. Genet. 17, 661–678 (2016). - PubMed
-
- Lieberman-Aiden E., van Berkum N. L., Williams L., Imakaev M., Ragoczy T., Telling A., Amit I., Lajoie B. R., Sabo P. J., Dorschner M. O., Sandstrom R., Bernstein B., Bender M. A., Groudine M., Gnirke A., Stamatoyannopoulos J., Mirny L. A., Lander E. S., Dekker J., Comprehensive mapping of long-range interactions reveals folding principles of the human genome. Science 326, 289–293 (2009). - PMC - PubMed
-
- Nora E. P., Lajoie B. R., Schulz E. G., Giorgetti L., Okamoto I., Servant N., Piolot T., van Berkum N. L., Meisig J., Sedat J., Gribnau J., Barillot E., Blüthgen N., Dekker J., Heard E., Spatial partitioning of the regulatory landscape of the X-inactivation centre. Nature 485, 381–385 (2012). - PMC - PubMed
Publication types
MeSH terms
Substances
LinkOut - more resources
Full Text Sources
Other Literature Sources
Molecular Biology Databases