The Yeast Saccharomyces cerevisiae as a Model for Understanding RAS Proteins and their Role in Human Tumorigenesis
- PMID: 29463063
- PMCID: PMC5850102
- DOI: 10.3390/cells7020014
The Yeast Saccharomyces cerevisiae as a Model for Understanding RAS Proteins and their Role in Human Tumorigenesis
Abstract
The exploitation of the yeast Saccharomyces cerevisiae as a biological model for the investigation of complex molecular processes conserved in multicellular organisms, such as humans, has allowed fundamental biological discoveries. When comparing yeast and human proteins, it is clear that both amino acid sequences and protein functions are often very well conserved. One example of the high degree of conservation between human and yeast proteins is highlighted by the members of the RAS family. Indeed, the study of the signaling pathways regulated by RAS in yeast cells led to the discovery of properties that were often found interchangeable with RAS proto-oncogenes in human pathways, and vice versa. In this work, we performed an updated critical literature review on human and yeast RAS pathways, specifically highlighting the similarities and differences between them. Moreover, we emphasized the contribution of studying yeast RAS pathways for the understanding of human RAS and how this model organism can contribute to unveil the roles of RAS oncoproteins in the regulation of mechanisms important in the tumorigenic process, like autophagy.
Keywords: KRAS; RAS proteins; S. cerevisiae; autophagy; colorectal cancer; homologues; model.
Conflict of interest statement
The authors declare no conflict of interest.
Figures
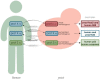
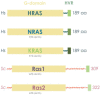
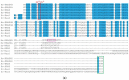
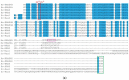
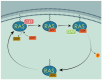
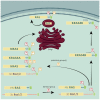
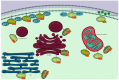
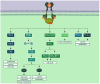
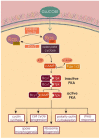
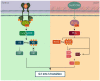
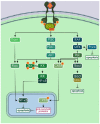
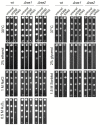
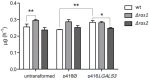
Similar articles
-
Exploring the function of RAS oncogenes by studying the yeast Saccharomyces cerevisiae.Princess Takamatsu Symp. 1986;17:253-60. Princess Takamatsu Symp. 1986. PMID: 3332013 Review.
-
Transmembrane signaling in Saccharomyces cerevisiae as a model for signaling in metazoans: state of the art after 25 years.Cell Signal. 2014 Dec;26(12):2865-78. doi: 10.1016/j.cellsig.2014.09.003. Epub 2014 Sep 15. Cell Signal. 2014. PMID: 25218923 Review.
-
Determinants of Ras proteins specifying the sensitivity to yeast Ira2p and human p120-GAP.EMBO J. 1996 Mar 1;15(5):1107-11. EMBO J. 1996. PMID: 8605880 Free PMC article.
-
The expanding role of yeast in cancer research and diagnosis: insights into the function of the oncosuppressors p53 and BRCA1/2.FEMS Yeast Res. 2014 Feb;14(1):2-16. doi: 10.1111/1567-1364.12094. Epub 2013 Oct 30. FEMS Yeast Res. 2014. PMID: 24103154 Review.
-
Regulation of autophagy in yeast Saccharomyces cerevisiae.Biochim Biophys Acta. 2009 Sep;1793(9):1413-21. doi: 10.1016/j.bbamcr.2009.01.008. Epub 2009 Jan 22. Biochim Biophys Acta. 2009. PMID: 19344676 Review.
Cited by
-
KRAS as a Modulator of the Inflammatory Tumor Microenvironment: Therapeutic Implications.Cells. 2022 Jan 24;11(3):398. doi: 10.3390/cells11030398. Cells. 2022. PMID: 35159208 Free PMC article. Review.
-
Rapid evolutionary repair by secondary perturbation of a primary disrupted transcriptional network.EMBO Rep. 2023 Jun 5;24(6):e56019. doi: 10.15252/embr.202256019. Epub 2023 Apr 3. EMBO Rep. 2023. PMID: 37009824 Free PMC article.
-
Analysis of RAS gene mutations in adverse events during first induction chemotherapy in childhood acute lymphoblastic leukemia.Transl Pediatr. 2023 Jan 31;12(1):56-67. doi: 10.21037/tp-22-683. Epub 2023 Jan 13. Transl Pediatr. 2023. PMID: 36798932 Free PMC article.
-
Specific Disruption of Ras2 CAAX Proteolysis Alters Its Localization and Function.Microbiol Spectr. 2023 Feb 14;11(1):e0269222. doi: 10.1128/spectrum.02692-22. Epub 2023 Jan 5. Microbiol Spectr. 2023. PMID: 36602340 Free PMC article.
-
The Cell Wall Integrity Receptor Mtl1 Contributes to Articulate Autophagic Responses When Glucose Availability Is Compromised.J Fungi (Basel). 2021 Oct 26;7(11):903. doi: 10.3390/jof7110903. J Fungi (Basel). 2021. PMID: 34829194 Free PMC article.
References
Publication types
LinkOut - more resources
Full Text Sources
Other Literature Sources
Molecular Biology Databases
Miscellaneous