Inhibition of GLO1 in Glioblastoma Multiforme Increases DNA-AGEs, Stimulates RAGE Expression, and Inhibits Brain Tumor Growth in Orthotopic Mouse Models
- PMID: 29385725
- PMCID: PMC5855628
- DOI: 10.3390/ijms19020406
Inhibition of GLO1 in Glioblastoma Multiforme Increases DNA-AGEs, Stimulates RAGE Expression, and Inhibits Brain Tumor Growth in Orthotopic Mouse Models
Abstract
Cancers that exhibit the Warburg effect may elevate expression of glyoxylase 1 (GLO1) to detoxify the toxic glycolytic byproduct methylglyoxal (MG) and inhibit the formation of pro-apoptotic advanced glycation endproducts (AGEs). Inhibition of GLO1 in cancers that up-regulate glycolysis has been proposed as a therapeutic targeting strategy, but this approach has not been evaluated for glioblastoma multiforme (GBM), the most aggressive and difficult to treat malignancy of the brain. Elevated GLO1 expression in GBM was established in patient tumors and cell lines using bioinformatics tools and biochemical approaches. GLO1 inhibition in GBM cell lines and in an orthotopic xenograft GBM mouse model was examined using both small molecule and short hairpin RNA (shRNA) approaches. Inhibition of GLO1 with S-(p-bromobenzyl) glutathione dicyclopentyl ester (p-BrBzGSH(Cp)₂) increased levels of the DNA-AGE N²-1-(carboxyethyl)-2'-deoxyguanosine (CEdG), a surrogate biomarker for nuclear MG exposure; substantially elevated expression of the immunoglobulin-like receptor for AGEs (RAGE); and induced apoptosis in GBM cell lines. Targeting GLO1 with shRNA similarly increased CEdG levels and RAGE expression, and was cytotoxic to glioma cells. Mice bearing orthotopic GBM xenografts treated systemically with p-BrBzGSH(Cp)₂ exhibited tumor regression without significant off-target effects suggesting that GLO1 inhibition may have value in the therapeutic management of these drug-resistant tumors.
Keywords: AGEs; CEdG; RAGE; glyoxalase 1; methylglyoxal.
Conflict of interest statement
The authors declare no conflict of interest.
Figures
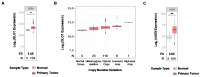
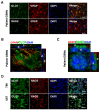
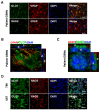
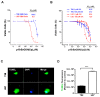
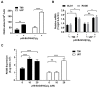
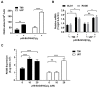
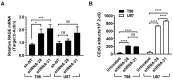
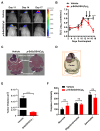
Similar articles
-
Emerging Glycation-Based Therapeutics-Glyoxalase 1 Inducers and Glyoxalase 1 Inhibitors.Int J Mol Sci. 2022 Feb 23;23(5):2453. doi: 10.3390/ijms23052453. Int J Mol Sci. 2022. PMID: 35269594 Free PMC article. Review.
-
Gene doubling increases glyoxalase 1 expression in RAGE knockout mice.Biochim Biophys Acta Gen Subj. 2020 Jan;1864(1):129438. doi: 10.1016/j.bbagen.2019.129438. Epub 2019 Sep 14. Biochim Biophys Acta Gen Subj. 2020. PMID: 31526867
-
Selective activation of apoptosis program by S-p-bromobenzylglutathione cyclopentyl diester in glyoxalase I-overexpressing human lung cancer cells.Clin Cancer Res. 2001 Aug;7(8):2513-8. Clin Cancer Res. 2001. PMID: 11489834
-
Anticancer drug candidate CBL0137, which inhibits histone chaperone FACT, is efficacious in preclinical orthotopic models of temozolomide-responsive and -resistant glioblastoma.Neuro Oncol. 2017 Feb 1;19(2):186-196. doi: 10.1093/neuonc/now141. Neuro Oncol. 2017. PMID: 27370399 Free PMC article.
-
Multiple roles of glyoxalase 1-mediated suppression of methylglyoxal glycation in cancer biology-Involvement in tumour suppression, tumour growth, multidrug resistance and target for chemotherapy.Semin Cancer Biol. 2018 Apr;49:83-93. doi: 10.1016/j.semcancer.2017.05.006. Epub 2017 May 12. Semin Cancer Biol. 2018. PMID: 28506645 Review.
Cited by
-
An Introduction to the Special Issue "Protein Glycation in Food, Nutrition, Health and Disease".Int J Mol Sci. 2022 Oct 27;23(21):13053. doi: 10.3390/ijms232113053. Int J Mol Sci. 2022. PMID: 36361833 Free PMC article.
-
Emerging Glycation-Based Therapeutics-Glyoxalase 1 Inducers and Glyoxalase 1 Inhibitors.Int J Mol Sci. 2022 Feb 23;23(5):2453. doi: 10.3390/ijms23052453. Int J Mol Sci. 2022. PMID: 35269594 Free PMC article. Review.
-
Leveraging histone glycation for cancer diagnostics and therapeutics.Trends Cancer. 2023 May;9(5):410-420. doi: 10.1016/j.trecan.2023.01.005. Epub 2023 Feb 18. Trends Cancer. 2023. PMID: 36804508 Free PMC article. Review.
-
Using AI-Based Evolutionary Algorithms to Elucidate Adult Brain Tumor (Glioma) Etiology Associated with IDH1 for Therapeutic Target Identification.Curr Issues Mol Biol. 2022 Jul 2;44(7):2982-3000. doi: 10.3390/cimb44070206. Curr Issues Mol Biol. 2022. PMID: 35877430 Free PMC article.
-
Methylglyoxal Acts as a Tumor-Promoting Factor in Anaplastic Thyroid Cancer.Cells. 2019 Jun 6;8(6):547. doi: 10.3390/cells8060547. Cells. 2019. PMID: 31174324 Free PMC article.
References
-
- Louis D.N., Perry A., Reifenberger G., von Deimling A., Figarella-Branger D., Cavenee W.K., Ohgaki H., Wiestler O.D., Kleihues P., Ellison D.W. The 2016 world health organization classification of tumors of the central nervous system: A summary. Acta Neuropathol. 2016;131:803–820. doi: 10.1007/s00401-016-1545-1. - DOI - PubMed
-
- Younes M., Lechago L.V., Somoano J.R., Mosharaf M., Lechago J. Wide expression of the human erythrocyte glucose transporter glut1 in human cancers. Cancer Res. 1996;56:1164–1167. - PubMed
MeSH terms
Substances
Grants and funding
LinkOut - more resources
Full Text Sources
Other Literature Sources
Medical
Research Materials
Miscellaneous