Cyclic Nucleotide-Directed Protein Kinases in Cardiovascular Inflammation and Growth
- PMID: 29367584
- PMCID: PMC5872354
- DOI: 10.3390/jcdd5010006
Cyclic Nucleotide-Directed Protein Kinases in Cardiovascular Inflammation and Growth
Abstract
Cardiovascular disease (CVD), including myocardial infarction (MI) and peripheral or coronary artery disease (PAD, CAD), remains the number one killer of individuals in the United States and worldwide, accounting for nearly 18 million (>30%) global deaths annually. Despite considerable basic science and clinical investigation aimed at identifying key etiologic components of and potential therapeutic targets for CVD, the number of individuals afflicted with these dreaded diseases continues to rise. Of the many biochemical, molecular, and cellular elements and processes characterized to date that have potential to control foundational facets of CVD, the multifaceted cyclic nucleotide pathways continue to be of primary basic science and clinical interest. Cyclic adenosine monophosphate (cyclic AMP) and cyclic guanosine monophosphate (cyclic GMP) and their plethora of downstream protein kinase effectors serve ubiquitous roles not only in cardiovascular homeostasis but also in the pathogenesis of CVD. Already a major target for clinical pharmacotherapy for CVD as well as other pathologies, novel and potentially clinically appealing actions of cyclic nucleotides and their downstream targets are still being discovered. With this in mind, this review article focuses on our current state of knowledge of the cyclic nucleotide-driven serine (Ser)/threonine (Thr) protein kinases in CVD with particular emphasis on cyclic AMP-dependent protein kinase (PKA) and cyclic GMP-dependent protein kinase (PKG). Attention is given to the regulatory interactions of these kinases with inflammatory components including interleukin 6 signals, with G protein-coupled receptor and growth factor signals, and with growth and synthetic transcriptional platforms underlying CVD pathogenesis. This article concludes with a brief discussion of potential future directions and highlights the importance for continued basic science and clinical study of cyclic nucleotide-directed protein kinases as emerging and crucial controllers of cardiac and vascular disease pathologies.
Keywords: G protein-coupled receptor; Smad3; Stat3; cyclic nucleotide; inflammation; interleukin 6; myocardial infarction; protease-activated receptor; protein kinase; vascular smooth muscle.
Conflict of interest statement
The authors declare no conflicts of interest.
Figures
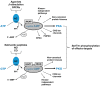
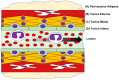
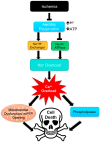
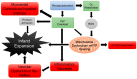
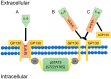
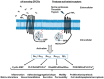
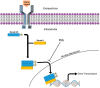
Similar articles
-
Soluble guanylyl cyclase-activated cyclic GMP-dependent protein kinase inhibits arterial smooth muscle cell migration independent of VASP-serine 239 phosphorylation.Cell Signal. 2016 Sep;28(9):1364-1379. doi: 10.1016/j.cellsig.2016.06.012. Epub 2016 Jun 11. Cell Signal. 2016. PMID: 27302407 Free PMC article.
-
ncRNAs and polyphenols: new therapeutic strategies for hypertension.RNA Biol. 2022;19(1):575-587. doi: 10.1080/15476286.2022.2066335. Epub 2021 Dec 31. RNA Biol. 2022. PMID: 35438046 Free PMC article.
-
Novel protein kinase targets in vascular smooth muscle therapeutics.Curr Opin Pharmacol. 2017 Apr;33:12-16. doi: 10.1016/j.coph.2017.03.003. Epub 2017 Apr 4. Curr Opin Pharmacol. 2017. PMID: 28388507 Free PMC article. Review.
-
The cGAS-STING signaling in cardiovascular and metabolic diseases: Future novel target option for pharmacotherapy.Acta Pharm Sin B. 2022 Jan;12(1):50-75. doi: 10.1016/j.apsb.2021.05.011. Epub 2021 May 20. Acta Pharm Sin B. 2022. PMID: 35127372 Free PMC article. Review.
-
Novel therapies for cyclic GMP control of vascular smooth muscle growth.Am J Ther. 2008 Nov-Dec;15(6):551-64. doi: 10.1097/MJT.0b013e318140052f. Am J Ther. 2008. PMID: 19127140 Free PMC article. Review.
Cited by
-
pH-sensing GPR68 inhibits vascular smooth muscle cell proliferation through Rap1A.Am J Physiol Heart Circ Physiol. 2024 Nov 1;327(5):H1210-H1229. doi: 10.1152/ajpheart.00413.2024. Epub 2024 Sep 13. Am J Physiol Heart Circ Physiol. 2024. PMID: 39269448
-
FoxO3 normalizes Smad3-induced arterial smooth muscle cell growth.Front Physiol. 2023 Aug 24;14:1136998. doi: 10.3389/fphys.2023.1136998. eCollection 2023. Front Physiol. 2023. PMID: 37693008 Free PMC article.
-
PDE-Mediated Cyclic Nucleotide Compartmentation in Vascular Smooth Muscle Cells: From Basic to a Clinical Perspective.J Cardiovasc Dev Dis. 2021 Dec 22;9(1):4. doi: 10.3390/jcdd9010004. J Cardiovasc Dev Dis. 2021. PMID: 35050214 Free PMC article. Review.
-
Therapeutic targeting of 3',5'-cyclic nucleotide phosphodiesterases: inhibition and beyond.Nat Rev Drug Discov. 2019 Oct;18(10):770-796. doi: 10.1038/s41573-019-0033-4. Epub 2019 Aug 6. Nat Rev Drug Discov. 2019. PMID: 31388135 Free PMC article. Review.
-
Protease-Activated Receptor 2 Controls Vascular Smooth Muscle Cell Proliferation in Cyclic AMP-Dependent Protein Kinase/Mitogen-Activated Protein Kinase Kinase 1/2-Dependent Manner.J Vasc Res. 2023;60(4):213-226. doi: 10.1159/000532032. Epub 2023 Sep 29. J Vasc Res. 2023. PMID: 37778342 Free PMC article.
References
-
- Benjamin E.J., Blaha M.J., Chiuve S.E., Cushman M., Das S.R., Deo R., de Ferranti S.D., Floyd J., Fornage M., Gillespie C., et al. Heart disease and stroke statistics-2017 update: A report from the American Heart Association. Circulation. 2017;135:e146–e603. doi: 10.1161/CIR.0000000000000485. - DOI - PMC - PubMed
-
- World Health Organization Cardiovascular Diseases (CVDs) Fact Sheet. [(accessed on 18 December 2017)]; Available online: http://www.who.int/mediacentre/factsheets/fs317/en/
-
- Adderley S.P., Joshi C.N., Martin D.N., Mooney S., Tulis D.A. Multiple kinase involvement in the regulation of vascular growth. In: Xavier G.D.S., editor. Advances in Protein Kinases. InTech Open Access Publishers; Rijeka, Croatia: 2012. pp. 131–150.
Publication types
Grants and funding
LinkOut - more resources
Full Text Sources
Other Literature Sources
Miscellaneous