Secreted Phospholipases A₂ from Animal Venoms in Pain and Analgesia
- PMID: 29311537
- PMCID: PMC5744126
- DOI: 10.3390/toxins9120406
Secreted Phospholipases A₂ from Animal Venoms in Pain and Analgesia
Abstract
Animal venoms comprise a complex mixture of components that affect several biological systems. Based on the high selectivity for their molecular targets, these components are also a rich source of potential therapeutic agents. Among the main components of animal venoms are the secreted phospholipases A₂ (sPLA₂s). These PLA₂ belong to distinct PLA₂s groups. For example, snake venom sPLA₂s from Elapidae and Viperidae families, the most important families when considering envenomation, belong, respectively, to the IA and IIA/IIB groups, whereas bee venom PLA₂ belongs to group III of sPLA₂s. It is well known that PLA₂, due to its hydrolytic activity on phospholipids, takes part in many pathophysiological processes, including inflammation and pain. Therefore, secreted PLA₂s obtained from animal venoms have been widely used as tools to (a) modulate inflammation and pain, uncovering molecular targets that are implicated in the control of inflammatory (including painful) and neurodegenerative diseases; (b) shed light on the pathophysiology of inflammation and pain observed in human envenomation by poisonous animals; and, (c) characterize molecular mechanisms involved in inflammatory diseases. The present review summarizes the knowledge on the nociceptive and antinociceptive actions of sPLA₂s from animal venoms, particularly snake venoms.
Keywords: analgesia; animal venoms; catalytic activity; pain; secretory phospholipases A2.
Conflict of interest statement
The authors declare no conflict of interest.
Figures
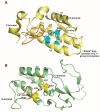
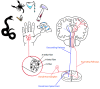
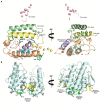
Similar articles
-
Snake venoms as a source of compounds modulating sperm physiology: Secreted phospholipases A2 from Oxyuranus scutellatus scutellatus impact sperm motility, acrosome reaction and in vitro fertilization in mice.Biochimie. 2010 Jul;92(7):826-36. doi: 10.1016/j.biochi.2010.03.003. Epub 2010 Mar 11. Biochimie. 2010. PMID: 20226834
-
Snake venomics and antivenomics of the arboreal neotropical pitvipers Bothriechis lateralis and Bothriechis schlegelii.J Proteome Res. 2008 Jun;7(6):2445-57. doi: 10.1021/pr8000139. Epub 2008 Apr 30. J Proteome Res. 2008. PMID: 18444672
-
Antibacterial activity of snake, scorpion and bee venoms: a comparison with purified venom phospholipase A2 enzymes.J Appl Microbiol. 2007 Mar;102(3):650-9. doi: 10.1111/j.1365-2672.2006.03161.x. J Appl Microbiol. 2007. PMID: 17309613
-
Antibacterial actions of secreted phospholipases A2. Review.Biochim Biophys Acta. 2008 Jan-Feb;1781(1-2):1-9. doi: 10.1016/j.bbalip.2007.12.001. Epub 2007 Dec 15. Biochim Biophys Acta. 2008. PMID: 18177747 Review.
-
Animal toxins as analgesics--an overview.Drug News Perspect. 2006 Sep;19(7):381-92. doi: 10.1358/dnp.2006.19.7.985940. Drug News Perspect. 2006. PMID: 17080201 Review.
Cited by
-
β-micrustoxin (Mlx-9), a PLA2 from Micrurus lemniscatus snake venom: biochemical characterization and anti-proliferative effect mediated by p53.J Venom Anim Toxins Incl Trop Dis. 2022 Apr 11;28:e20210094. doi: 10.1590/1678-9199-JVATITD-2021-0094. eCollection 2022. J Venom Anim Toxins Incl Trop Dis. 2022. PMID: 35432496 Free PMC article.
-
Crosstalk of Inflammation and Coagulation in Bothrops Snakebite Envenoming: Endogenous Signaling Pathways and Pathophysiology.Int J Mol Sci. 2023 Jul 15;24(14):11508. doi: 10.3390/ijms241411508. Int J Mol Sci. 2023. PMID: 37511277 Free PMC article. Review.
-
Effect of Zhongyi paste on inflammatory pain in mice by regulation of the extracellular regulated protein kinases 1/2-cyclooxygenase-2-prostaglandin E2 pathway.Korean J Pain. 2020 Oct 1;33(4):335-343. doi: 10.3344/kjp.2020.33.4.335. Korean J Pain. 2020. PMID: 32989198 Free PMC article.
-
Stings on wings: Proteotranscriptomic and biochemical profiling of the lesser banded hornet (Vespa affinis) venom.Front Mol Biosci. 2022 Dec 19;9:1066793. doi: 10.3389/fmolb.2022.1066793. eCollection 2022. Front Mol Biosci. 2022. PMID: 36601583 Free PMC article.
-
Evaluation of the Inhibitory Potential of Casuarictin, an Ellagitannin Isolated from White Mangrove (Laguncularia racemosa) Leaves, on Snake Venom Secretory Phospholipase A2.Mar Drugs. 2019 Jul 8;17(7):403. doi: 10.3390/md17070403. Mar Drugs. 2019. PMID: 31288445 Free PMC article.
References
Publication types
MeSH terms
Substances
LinkOut - more resources
Full Text Sources
Other Literature Sources
Medical