Phosphoinositide conversion in endocytosis and the endolysosomal system
- PMID: 29282290
- PMCID: PMC5798284
- DOI: 10.1074/jbc.R117.000629
Phosphoinositide conversion in endocytosis and the endolysosomal system
Abstract
Phosphoinositides (PIs) are phospholipids that perform crucial cell functions, ranging from cell migration and signaling to membrane trafficking, by serving as signposts of compartmental membrane identity. Although phosphatidylinositol 4,5-bisphosphate, 3-phosphate, and 3,5-bisphosphate are commonly considered as hallmarks of the plasma membrane, endosomes, and lysosomes, these compartments contain other functionally important PIs. Here, we review the roles of PIs in different compartments of the endolysosomal system in mammalian cells and discuss the mechanisms that spatiotemporally control PI conversion in endocytosis and endolysosomal membrane dynamics during endosome maturation and sorting. As defective PI conversion underlies human genetic diseases, including inherited myopathies, neurological disorders, and cancer, PI-converting enzymes represent potential targets for drug-based therapies.
Keywords: autophagy; endocytosis; endosome; lysosome; mTOR complex (mTORC); phosphatidylinositol; phosphatidylinositol kinase (PI Kinase); phosphatidylinositol phosphatase; phosphatidylinositol signaling.
© 2018 by The American Society for Biochemistry and Molecular Biology, Inc.
Conflict of interest statement
The authors declare that they have no conflicts of interest with the contents of this article
Figures
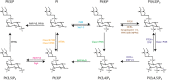
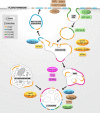
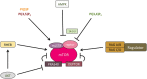
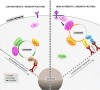
Similar articles
-
A phosphoinositide conversion mechanism for exit from endosomes.Nature. 2016 Jan 21;529(7586):408-12. doi: 10.1038/nature16516. Epub 2016 Jan 13. Nature. 2016. PMID: 26760201
-
Phosphatidylinositol 3,4-bisphosphate synthesis and turnover are spatially segregated in the endocytic pathway.J Biol Chem. 2020 Jan 24;295(4):1091-1104. doi: 10.1074/jbc.RA119.011774. Epub 2019 Dec 12. J Biol Chem. 2020. PMID: 31831620 Free PMC article.
-
Phosphoinositides in endocytosis.Biochim Biophys Acta. 2015 Jun;1851(6):794-804. doi: 10.1016/j.bbalip.2014.09.014. Epub 2014 Sep 28. Biochim Biophys Acta. 2015. PMID: 25264171 Review.
-
Phosphoinositide switches in endocytosis and in the endolysosomal system.Curr Opin Cell Biol. 2019 Aug;59:50-57. doi: 10.1016/j.ceb.2019.03.011. Epub 2019 Apr 28. Curr Opin Cell Biol. 2019. PMID: 31029845 Review.
-
Sequential conversion of PtdIns3P to PtdIns(3,5)P2 via endosome maturation couples nutrient signaling to lysosome reformation and basal autophagy.Autophagy. 2023 Apr;19(4):1365-1367. doi: 10.1080/15548627.2022.2124499. Epub 2022 Sep 26. Autophagy. 2023. PMID: 36103410 Free PMC article.
Cited by
-
Phosphoinositide lipids in primary cilia biology.Biochem J. 2020 Sep 30;477(18):3541-3565. doi: 10.1042/BCJ20200277. Biochem J. 2020. PMID: 32970140 Free PMC article. Review.
-
Small molecules targeting endocytic uptake and recycling pathways.Front Cell Dev Biol. 2023 Mar 10;11:1125801. doi: 10.3389/fcell.2023.1125801. eCollection 2023. Front Cell Dev Biol. 2023. PMID: 36968200 Free PMC article. Review.
-
Mechanisms Associated with Trypanosoma cruzi Host Target Cell Adhesion, Recognition and Internalization.Life (Basel). 2021 Jun 9;11(6):534. doi: 10.3390/life11060534. Life (Basel). 2021. PMID: 34207491 Free PMC article. Review.
-
Held Up in Traffic-Defects in the Trafficking Machinery in Charcot-Marie-Tooth Disease.Front Mol Neurosci. 2021 Aug 16;14:695294. doi: 10.3389/fnmol.2021.695294. eCollection 2021. Front Mol Neurosci. 2021. PMID: 34483837 Free PMC article. Review.
-
Voltage-sensing phosphatase (Vsp) regulates endocytosis-dependent nutrient absorption in chordate enterocytes.Commun Biol. 2022 Sep 10;5(1):948. doi: 10.1038/s42003-022-03916-6. Commun Biol. 2022. PMID: 36088390 Free PMC article.
References
-
- Baskin J. M., Wu X., Christiano R., Oh M. S., Schauder C. M., Gazzerro E., Messa M., Baldassari S., Assereto S., Biancheri R., Zara F., Minetti C., Raimondi A., Simons M., Walther T. C., et al. (2016) The leukodystrophy protein FAM126A (hyccin) regulates PtdIns(4)P synthesis at the plasma membrane. Nat. Cell Biol. 18, 132–138 10.1038/ncb3271 - DOI - PMC - PubMed
Publication types
MeSH terms
Substances
LinkOut - more resources
Full Text Sources
Other Literature Sources
Research Materials
Miscellaneous