Regulation of Carbohydrate Energy Metabolism in Drosophila melanogaster
- PMID: 29203701
- PMCID: PMC5714444
- DOI: 10.1534/genetics.117.199885
Regulation of Carbohydrate Energy Metabolism in Drosophila melanogaster
Abstract
Carbohydrate metabolism is essential for cellular energy balance as well as for the biosynthesis of new cellular building blocks. As animal nutrient intake displays temporal fluctuations and each cell type within the animal possesses specific metabolic needs, elaborate regulatory systems are needed to coordinate carbohydrate metabolism in time and space. Carbohydrate metabolism is regulated locally through gene regulatory networks and signaling pathways, which receive inputs from nutrient sensors as well as other pathways, such as developmental signals. Superimposed on cell-intrinsic control, hormonal signaling mediates intertissue information to maintain organismal homeostasis. Misregulation of carbohydrate metabolism is causative for many human diseases, such as diabetes and cancer. Recent work in Drosophila melanogaster has uncovered new regulators of carbohydrate metabolism and introduced novel physiological roles for previously known pathways. Moreover, genetically tractable Drosophila models to study carbohydrate metabolism-related human diseases have provided new insight into the mechanisms of pathogenesis. Due to the high degree of conservation of relevant regulatory pathways, as well as vast possibilities for the analysis of gene-nutrient interactions and tissue-specific gene function, Drosophila is emerging as an important model system for research on carbohydrate metabolism.
Keywords: gene regulation; glucose; insulin; metabolism; nutrient sensing.
Copyright © 2017 by the Genetics Society of America.
Figures
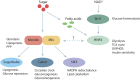
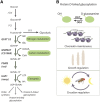
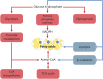
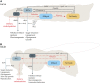
Similar articles
-
Physiological Adaptations to Sugar Intake: New Paradigms from Drosophila melanogaster.Trends Endocrinol Metab. 2017 Feb;28(2):131-142. doi: 10.1016/j.tem.2016.11.003. Epub 2016 Dec 5. Trends Endocrinol Metab. 2017. PMID: 27923532 Review.
-
Modeling metabolic homeostasis and nutrient sensing in Drosophila: implications for aging and metabolic diseases.Dis Model Mech. 2014 Mar;7(3):343-50. doi: 10.1242/dmm.012989. Dis Model Mech. 2014. PMID: 24609035 Free PMC article. Review.
-
The epicurean fly: using Drosophila melanogaster to study metabolism.Pediatr Res. 2009 Feb;65(2):132-7. doi: 10.1203/PDR.0b013e318191fc68. Pediatr Res. 2009. PMID: 18978647 Review.
-
Discovering signaling mechanisms governing metabolism and metabolic diseases with Drosophila.Cell Metab. 2021 Jul 6;33(7):1279-1292. doi: 10.1016/j.cmet.2021.05.018. Epub 2021 Jun 16. Cell Metab. 2021. PMID: 34139200 Free PMC article. Review.
-
Tipping the metabolic scales towards increased longevity in mammals.Nat Cell Biol. 2015 Mar;17(3):196-203. doi: 10.1038/ncb3107. Nat Cell Biol. 2015. PMID: 25720959 Review.
Cited by
-
Disruption of the Serine/Threonine Kinase Akt Gene Affects Ovarian Development and Fecundity in the Cigarette Beetle, Lasioderma serricorne.Front Physiol. 2021 Oct 7;12:765819. doi: 10.3389/fphys.2021.765819. eCollection 2021. Front Physiol. 2021. PMID: 34690822 Free PMC article.
-
Antiaging function of Chinese pond turtle (Chinemys reevesii) peptide through activation of the Nrf2/Keap1 signaling pathway and its structure-activity relationship.Front Nutr. 2022 Jul 22;9:961922. doi: 10.3389/fnut.2022.961922. eCollection 2022. Front Nutr. 2022. PMID: 35938097 Free PMC article.
-
A cis-regulatory element promoting increased transcription at low temperature in cultured ectothermic Drosophila cells.BMC Genomics. 2021 Oct 28;22(1):771. doi: 10.1186/s12864-021-08057-4. BMC Genomics. 2021. PMID: 34711176 Free PMC article.
-
Mechanisms of Neuroendocrine Stress Response in Drosophila and Its Effect on Carbohydrate and Lipid Metabolism.Insects. 2023 May 17;14(5):474. doi: 10.3390/insects14050474. Insects. 2023. PMID: 37233102 Free PMC article. Review.
-
Regulatory Functions of Nilaparvata lugens GSK-3 in Energy and Chitin Metabolism.Front Physiol. 2020 Nov 25;11:518876. doi: 10.3389/fphys.2020.518876. eCollection 2020. Front Physiol. 2020. PMID: 33324230 Free PMC article.
References
Publication types
MeSH terms
Substances
LinkOut - more resources
Full Text Sources
Medical
Molecular Biology Databases
Research Materials