A lysosomal switch triggers proteostasis renewal in the immortal C. elegans germ lineage
- PMID: 29168500
- PMCID: PMC5936623
- DOI: 10.1038/nature24620
A lysosomal switch triggers proteostasis renewal in the immortal C. elegans germ lineage
Erratum in
-
Addendum: A lysosomal switch triggers proteostasis renewal in the immortal C. elegans germ lineage.Nature. 2020 Apr;580(7802):E5. doi: 10.1038/s41586-020-2108-0. Nature. 2020. PMID: 32269336 No abstract available.
Abstract
Although individuals age and die with time, an animal species can continue indefinitely, because of its immortal germ-cell lineage. How the germline avoids transmitting damage from one generation to the next remains a fundamental question in biology. Here we identify a lysosomal switch that enhances germline proteostasis before fertilization. We find that Caenorhabditis elegans oocytes whose maturation is arrested by the absence of sperm exhibit hallmarks of proteostasis collapse, including protein aggregation. Remarkably, sperm-secreted hormones re-establish oocyte proteostasis once fertilization becomes imminent. Key to this restoration is activation of the vacuolar H+-ATPase (V-ATPase), a proton pump that acidifies lysosomes. Sperm stimulate V-ATPase activity in oocytes by signalling the degradation of GLD-1, a translational repressor that blocks V-ATPase synthesis. Activated lysosomes, in turn, promote a metabolic shift that mobilizes protein aggregates for degradation, and reset proteostasis by enveloping and clearing the aggregates. Lysosome acidification also occurs during Xenopus oocyte maturation; thus, a lysosomal switch that enhances oocyte proteostasis in anticipation of fertilization may be conserved in other species.
Figures
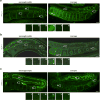
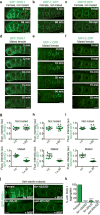
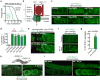
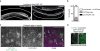
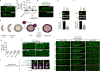
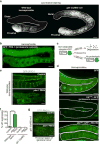
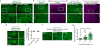
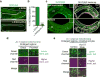
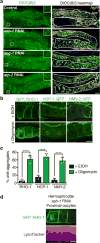
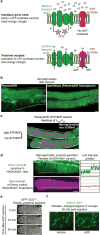
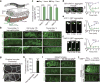
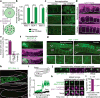
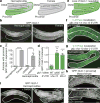
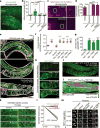
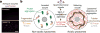
Comment in
-
Putting Your Best Egg Forward.Dev Cell. 2018 Jan 8;44(1):5-7. doi: 10.1016/j.devcel.2017.12.016. Epub 2018 Jan 8. Dev Cell. 2018. PMID: 29316440 Free PMC article.
-
No Excess Baggage: New Life Starts with a Clean Slate.Mol Cell. 2018 Jan 18;69(2):163-164. doi: 10.1016/j.molcel.2017.12.033. Mol Cell. 2018. PMID: 29351841
Similar articles
-
A genetic screen identifies new steps in oocyte maturation that enhance proteostasis in the immortal germ lineage.Elife. 2021 Apr 13;10:e62653. doi: 10.7554/eLife.62653. Elife. 2021. PMID: 33848238 Free PMC article.
-
MPK-1/ERK regulatory network controls the number of sperm by regulating timing of sperm-oocyte switch in C. elegans germline.Biochem Biophys Res Commun. 2017 Sep 30;491(4):1077-1082. doi: 10.1016/j.bbrc.2017.08.014. Epub 2017 Aug 3. Biochem Biophys Res Commun. 2017. PMID: 28782521 Free PMC article.
-
GLS-1, a novel P granule component, modulates a network of conserved RNA regulators to influence germ cell fate decisions.PLoS Genet. 2009 May;5(5):e1000494. doi: 10.1371/journal.pgen.1000494. Epub 2009 May 22. PLoS Genet. 2009. PMID: 19461891 Free PMC article.
-
Role of PUF-8/PUF protein in stem cell control, sperm-oocyte decision and cell fate reprogramming.J Cell Physiol. 2014 Oct;229(10):1306-11. doi: 10.1002/jcp.24618. J Cell Physiol. 2014. PMID: 24638209 Review.
-
Control of oocyte meiotic maturation and fertilization.WormBook. 2005 Dec 28:1-12. doi: 10.1895/wormbook.1.53.1. WormBook. 2005. PMID: 18050412 Free PMC article. Review.
Cited by
-
Mechanical force of uterine occupation enables large vesicle extrusion from proteostressed maternal neurons.Elife. 2024 Sep 10;13:RP95443. doi: 10.7554/eLife.95443. Elife. 2024. PMID: 39255003 Free PMC article.
-
The HEAT repeat protein HPO-27 is a lysosome fission factor.Nature. 2024 Apr;628(8008):630-638. doi: 10.1038/s41586-024-07249-8. Epub 2024 Mar 27. Nature. 2024. PMID: 38538795
-
The Ground Zero of Organismal Life and Aging.Trends Mol Med. 2021 Jan;27(1):11-19. doi: 10.1016/j.molmed.2020.08.012. Epub 2020 Sep 23. Trends Mol Med. 2021. PMID: 32980264 Free PMC article. Review.
-
A genetic screen identifies new steps in oocyte maturation that enhance proteostasis in the immortal germ lineage.Elife. 2021 Apr 13;10:e62653. doi: 10.7554/eLife.62653. Elife. 2021. PMID: 33848238 Free PMC article.
-
Oocyte aging is controlled by mitogen-activated protein kinase signaling.Aging Cell. 2021 Jun;20(6):e13386. doi: 10.1111/acel.13386. Epub 2021 Jun 1. Aging Cell. 2021. PMID: 34061407 Free PMC article.
References
-
- Medvedev ZA. On the immortality of the germ line: genetic and biochemical mechanism. Mech Ageing Dev. 1981;17:331–359. - PubMed
-
- Miller MA, et al. A sperm cytoskeletal protein that signals oocyte meiotic maturation and ovulation. Science. 2001;291:2144–2147. - PubMed
-
- Nousch M, Eckmann CR. Translational control in the Caenorhabditis elegans germ line. Adv Exp Med Biol. 2013;757:205–247. - PubMed
-
- Goudeau J, Aguilaniu H. Carbonylated proteins are eliminated during reproduction in C. elegans. Aging Cell. 2010;9:991–1003. - PubMed
Publication types
MeSH terms
Substances
Grants and funding
LinkOut - more resources
Full Text Sources
Other Literature Sources
Molecular Biology Databases