Coordinated Regulation of Hypocotyl Cell Elongation by Light and Ethylene through a Microtubule Destabilizing Protein
- PMID: 29167353
- PMCID: PMC5761786
- DOI: 10.1104/pp.17.01109
Coordinated Regulation of Hypocotyl Cell Elongation by Light and Ethylene through a Microtubule Destabilizing Protein
Abstract
Precise regulation of hypocotyl cell elongation is essential for plant growth and survival. Light suppresses hypocotyl elongation by degrading transcription factor phytochrome-interacting factor 3 (PIF3), whereas the phytohormone ethylene promotes hypocotyl elongation by activating PIF3. However, the underlying mechanisms regarding how these two pathways coordinate downstream effectors to mediate hypocotyl elongation are largely unclear. In this study, we identified the novel Microtubule-Destabilizing Protein 60 (MDP60), which plays a positive role in hypocotyl cell elongation in Arabidopsis (Arabidopsis thaliana); this effect is mediated through PIF3. Ethylene signaling up-regulates MDP60 expression via PIF3 binding to the MDP60 promoter. MDP60 loss-of-function mutants exhibit much shorter hypocotyls, whereas MDP60 overexpression significantly promotes hypocotyl cell elongation when grown in light compared to the control. MDP60 protein binds to microtubules in vitro and in vivo. The organization of cortical microtubules was significantly disrupted in mdp60 mutant cells and MDP60-overexpressing seedlings. These findings indicate that MDP60 is an important mediator of hypocotyl cell elongation. This study reveals a mechanism in which light and ethylene signaling coordinate MDP60 expression to modulate hypocotyl cell elongation by altering cortical microtubules in Arabidopsis.
© 2018 American Society of Plant Biologists. All Rights Reserved.
Figures
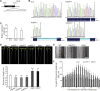
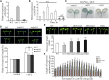
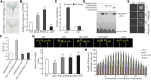
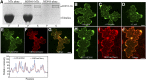
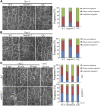
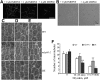
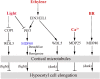
Similar articles
-
Submergence stress-induced hypocotyl elongation through ethylene signaling-mediated regulation of cortical microtubules in Arabidopsis.J Exp Bot. 2020 Jan 23;71(3):1067-1077. doi: 10.1093/jxb/erz453. J Exp Bot. 2020. PMID: 31638649
-
Ethylene Regulates the Arabidopsis Microtubule-Associated Protein WAVE-DAMPENED2-LIKE5 in Etiolated Hypocotyl Elongation.Plant Physiol. 2015 Sep;169(1):325-37. doi: 10.1104/pp.15.00609. Epub 2015 Jul 1. Plant Physiol. 2015. PMID: 26134166 Free PMC article.
-
Thermoperiodic control of hypocotyl elongation depends on auxin-induced ethylene signaling that controls downstream PHYTOCHROME INTERACTING FACTOR3 activity.Plant Physiol. 2015 Feb;167(2):517-30. doi: 10.1104/pp.114.254425. Epub 2014 Dec 16. Plant Physiol. 2015. PMID: 25516603 Free PMC article.
-
The Arabidopsis thaliana hypocotyl, a model to identify and study control mechanisms of cellular expansion.Plant Cell Rep. 2014 May;33(5):697-706. doi: 10.1007/s00299-014-1591-x. Epub 2014 Mar 16. Plant Cell Rep. 2014. PMID: 24633990 Review.
-
Integration of Ethylene and Light Signaling Affects Hypocotyl Growth in Arabidopsis.Front Plant Sci. 2017 Jan 24;8:57. doi: 10.3389/fpls.2017.00057. eCollection 2017. Front Plant Sci. 2017. PMID: 28174592 Free PMC article. Review.
Cited by
-
Regulation of Plant Photoresponses by Protein Kinase Activity of Phytochrome A.Int J Mol Sci. 2023 Jan 20;24(3):2110. doi: 10.3390/ijms24032110. Int J Mol Sci. 2023. PMID: 36768431 Free PMC article. Review.
-
Exogenous Serotonin (5-HT) Promotes Mesocotyl and Coleoptile Elongation in Maize Seedlings under Deep-Seeding Stress through Enhancing Auxin Accumulation and Inhibiting Lignin Formation.Int J Mol Sci. 2023 Dec 2;24(23):17061. doi: 10.3390/ijms242317061. Int J Mol Sci. 2023. PMID: 38069387 Free PMC article.
-
A dual mode of ethylene actions contributes to the optimization of hypocotyl growth under fluctuating temperature environments.Plant Signal Behav. 2021 Sep 2;16(9):1926131. doi: 10.1080/15592324.2021.1926131. Epub 2021 May 11. Plant Signal Behav. 2021. PMID: 33975509 Free PMC article.
-
Ethylene Signaling Modulates Cortical Microtubule Reassembly in Response to Salt Stress.Plant Physiol. 2018 Mar;176(3):2071-2081. doi: 10.1104/pp.17.01124. Epub 2018 Feb 5. Plant Physiol. 2018. PMID: 29431630 Free PMC article.
-
GR24, A Synthetic Strigolactone Analog, and Light Affect the Organization of Cortical Microtubules in Arabidopsis Hypocotyl Cells.Front Plant Sci. 2021 Jul 7;12:675981. doi: 10.3389/fpls.2021.675981. eCollection 2021. Front Plant Sci. 2021. PMID: 34305975 Free PMC article.
References
-
- Alonso JM, Hirayama T, Roman G, Nourizadeh S, Ecker JR (1999) EIN2, a bifunctional transducer of ethylene and stress responses in Arabidopsis. Science 284: 2148–2152 - PubMed
-
- Al-Sady B, Ni W, Kircher S, Schäfer E, Quail PH (2006) Photoactivated phytochrome induces rapid PIF3 phosphorylation prior to proteasome-mediated degradation. Mol Cell 23: 439–446 - PubMed
-
- Castillon A, Shen H, Huq E (2007) Phytochrome Interacting Factors: central players in phytochrome-mediated light signaling networks. Trends Plant Sci 12: 514–521 - PubMed
Publication types
MeSH terms
Substances
LinkOut - more resources
Full Text Sources
Other Literature Sources
Molecular Biology Databases
Research Materials