Yeast Killer Toxin K28: Biology and Unique Strategy of Host Cell Intoxication and Killing
- PMID: 29053588
- PMCID: PMC5666379
- DOI: 10.3390/toxins9100333
Yeast Killer Toxin K28: Biology and Unique Strategy of Host Cell Intoxication and Killing
Erratum in
-
Correction: Becker, B. et al. Yeast Killer Toxin K28: Biology and Unique Strategy of Host Cell Intoxication and Killing.Toxins (Basel). 2018 Mar 23;10(4):132. doi: 10.3390/toxins10040132. Toxins (Basel). 2018. PMID: 29570623 Free PMC article. No abstract available.
Abstract
The initial discovery of killer toxin-secreting brewery strains of Saccharomyces cerevisiae (S. cerevisiae) in the mid-sixties of the last century marked the beginning of intensive research in the yeast virology field. So far, four different S. cerevisiae killer toxins (K28, K1, K2, and Klus), encoded by cytoplasmic inherited double-stranded RNA viruses (dsRNA) of the Totiviridae family, have been identified. Among these, K28 represents the unique example of a yeast viral killer toxin that enters a sensitive cell by receptor-mediated endocytosis to reach its intracellular target(s). This review summarizes and discusses the most recent advances and current knowledge on yeast killer toxin K28, with special emphasis on its endocytosis and intracellular trafficking, pointing towards future directions and open questions in this still timely and fascinating field of killer yeast research.
Keywords: A/B toxin; H/KDEL receptor; K28; S. cerevisiae; cell cycle arrest; cell wall receptor; killer toxin; retrograde protein transport; retrotranslocation; toxin immunity.
Conflict of interest statement
The authors declare no conflict of interest.
Figures
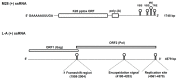
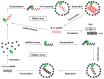
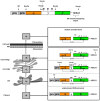

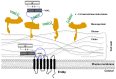
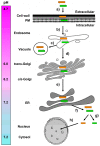
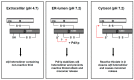
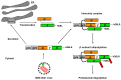
Similar articles
-
Production of fluorescent and cytotoxic K28 killer toxin variants through high cell density fermentation of recombinant Pichia pastoris.Microb Cell Fact. 2017 Dec 19;16(1):228. doi: 10.1186/s12934-017-0844-0. Microb Cell Fact. 2017. PMID: 29258515 Free PMC article.
-
Relationships and Evolution of Double-Stranded RNA Totiviruses of Yeasts Inferred from Analysis of L-A-2 and L-BC Variants in Wine Yeast Strain Populations.Appl Environ Microbiol. 2017 Feb 1;83(4):e02991-16. doi: 10.1128/AEM.02991-16. Print 2017 Feb 15. Appl Environ Microbiol. 2017. PMID: 27940540 Free PMC article.
-
A yeast killer toxin screen provides insights into a/b toxin entry, trafficking, and killing mechanisms.Dev Cell. 2009 Oct;17(4):552-60. doi: 10.1016/j.devcel.2009.08.006. Dev Cell. 2009. PMID: 19853568 Free PMC article.
-
Viral induced yeast apoptosis.Biochim Biophys Acta. 2008 Jul;1783(7):1413-7. doi: 10.1016/j.bbamcr.2008.01.017. Epub 2008 Feb 7. Biochim Biophys Acta. 2008. PMID: 18291112 Review.
-
Yeast dsRNA viruses: replication and killer phenotypes.Mol Microbiol. 1991 Oct;5(10):2331-8. doi: 10.1111/j.1365-2958.1991.tb02078.x. Mol Microbiol. 1991. PMID: 1665194 Review.
Cited by
-
The prevalence of killer yeasts and double-stranded RNAs in the budding yeast Saccharomyces cerevisiae.FEMS Yeast Res. 2023 Jan 4;23:foad046. doi: 10.1093/femsyr/foad046. FEMS Yeast Res. 2023. PMID: 37935474 Free PMC article.
-
Molecular and Physiological Diversity of Indigenous Yeasts Isolated from Spontaneously Fermented Wine Wort from Ilfov County, Romania.Microorganisms. 2022 Dec 22;11(1):37. doi: 10.3390/microorganisms11010037. Microorganisms. 2022. PMID: 36677329 Free PMC article.
-
Genome sequence of the non-conventional wine yeast Hanseniaspora guilliermondii UTAD222 unveils relevant traits of this species and of the Hanseniaspora genus in the context of wine fermentation.DNA Res. 2019 Feb 1;26(1):67-83. doi: 10.1093/dnares/dsy039. DNA Res. 2019. PMID: 30462193 Free PMC article.
-
The evolutionary ecology of fungal killer phenotypes.Proc Biol Sci. 2023 Aug 30;290(2005):20231108. doi: 10.1098/rspb.2023.1108. Epub 2023 Aug 16. Proc Biol Sci. 2023. PMID: 37583325 Free PMC article. Review.
-
Occurrence of Yeasts in White-Brined Cheeses: Methodologies for Identification, Spoilage Potential and Good Manufacturing Practices.Front Microbiol. 2020 Oct 15;11:582778. doi: 10.3389/fmicb.2020.582778. eCollection 2020. Front Microbiol. 2020. PMID: 33178163 Free PMC article. Review.
References
-
- Bevan E.A., Makower M. The physiological basis of the killer character in yeast; Proceedings of the 11th International Congress Genet; The Hague, The Netherlands. September 1963; pp. 202–203.
-
- Theisen S., Molkenau E., Schmitt M.J. Wicaltin, a new protein toxin secreted by the yeast williopsis californica and its broad-spectrum antimycotic potential. J. Microbiol. Biotechnol. 2000;10:547–550.
Publication types
MeSH terms
Substances
LinkOut - more resources
Full Text Sources
Other Literature Sources
Molecular Biology Databases