Combination of Cα-H Hydrogen Bonds and van der Waals Packing Modulates the Stability of GxxxG-Mediated Dimers in Membranes
- PMID: 29028318
- PMCID: PMC5927632
- DOI: 10.1021/jacs.7b07505
Combination of Cα-H Hydrogen Bonds and van der Waals Packing Modulates the Stability of GxxxG-Mediated Dimers in Membranes
Abstract
The GxxxG motif is frequently found at the dimerization interface of a transmembrane structural motif called GASright, which is characterized by a short interhelical distance and a right-handed crossing angle between the helices. In GASright dimers, such as glycophorin A (GpA), BNIP3, and members of the ErbB family, the backbones of the helices are in contact, and they invariably display networks of 4 to 8 weak hydrogen bonds between Cα-H carbon donors and carbonyl acceptors on opposing helices (Cα-H···O═C hydrogen bonds). These networks of weak hydrogen bonds at the helix-helix interface are presumably stabilizing, but their energetic contribution to dimerization has yet to be determined experimentally. Here, we present a computational and experimental structure-based analysis of GASright dimers of different predicted stabilities, which show that a combination of van der Waals packing and Cα-H hydrogen bonding predicts the experimental trend of dimerization propensities. This finding provides experimental support for the hypothesis that the networks of Cα-H hydrogen bonds are major contributors to the free energy of association of GxxxG-mediated dimers. The structural comparison between groups of GASright dimers of different stabilities reveals distinct sequence as well as conformational preferences. Stability correlates with shorter interhelical distances, narrower crossing angles, better packing, and the formation of larger networks of Cα-H hydrogen bonds. The identification of these structural rules provides insight on how nature could modulate stability in GASright and finely tune dimerization to support biological function.
Conflict of interest statement
The authors declare no competing financial interest.
Figures
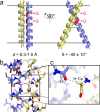
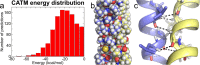
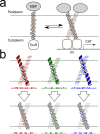
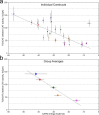
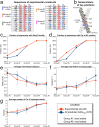
Similar articles
-
Thermodynamic analysis of the GASright transmembrane motif supports energetic model of dimerization.Biophys J. 2023 Jan 3;122(1):143-155. doi: 10.1016/j.bpj.2022.11.018. Epub 2022 Nov 12. Biophys J. 2023. PMID: 36371634 Free PMC article.
-
A frequent, GxxxG-mediated, transmembrane association motif is optimized for the formation of interhelical Cα-H hydrogen bonds.Proc Natl Acad Sci U S A. 2014 Mar 11;111(10):E888-95. doi: 10.1073/pnas.1319944111. Epub 2014 Feb 25. Proc Natl Acad Sci U S A. 2014. PMID: 24569864 Free PMC article.
-
Sequence dependence of BNIP3 transmembrane domain dimerization implicates side-chain hydrogen bonding and a tandem GxxxG motif in specific helix-helix interactions.J Mol Biol. 2006 Dec 15;364(5):974-90. doi: 10.1016/j.jmb.2006.09.065. Epub 2006 Sep 29. J Mol Biol. 2006. PMID: 17049556
-
Role of GxxxG Motifs in Transmembrane Domain Interactions.Biochemistry. 2015 Aug 25;54(33):5125-35. doi: 10.1021/acs.biochem.5b00495. Epub 2015 Aug 13. Biochemistry. 2015. PMID: 26244771 Review.
-
Sequence motifs, polar interactions and conformational changes in helical membrane proteins.Curr Opin Struct Biol. 2003 Aug;13(4):412-7. doi: 10.1016/s0959-440x(03)00102-7. Curr Opin Struct Biol. 2003. PMID: 12948770 Review.
Cited by
-
Packing of apolar side chains enables accurate design of highly stable membrane proteins.Science. 2019 Mar 29;363(6434):1418-1423. doi: 10.1126/science.aav7541. Science. 2019. PMID: 30923216 Free PMC article.
-
Folding and Misfolding of Human Membrane Proteins in Health and Disease: From Single Molecules to Cellular Proteostasis.Chem Rev. 2019 May 8;119(9):5537-5606. doi: 10.1021/acs.chemrev.8b00532. Epub 2019 Jan 4. Chem Rev. 2019. PMID: 30608666 Free PMC article.
-
Small Residues Inhibit Homo-Dimerization of the Human Carbonic Anhydrase XII Transmembrane Domain.Membranes (Basel). 2021 Jul 7;11(7):512. doi: 10.3390/membranes11070512. Membranes (Basel). 2021. PMID: 34357162 Free PMC article.
-
Thermodynamic analysis of the GASright transmembrane motif supports energetic model of dimerization.Biophys J. 2023 Jan 3;122(1):143-155. doi: 10.1016/j.bpj.2022.11.018. Epub 2022 Nov 12. Biophys J. 2023. PMID: 36371634 Free PMC article.
-
Membrane receptor activation mechanisms and transmembrane peptide tools to elucidate them.J Biol Chem. 2020 Feb 14;295(7):1792-1814. doi: 10.1074/jbc.REV119.009457. Epub 2019 Dec 25. J Biol Chem. 2020. PMID: 31879273 Free PMC article. Review.
References
Publication types
MeSH terms
Substances
Grants and funding
LinkOut - more resources
Full Text Sources
Other Literature Sources
Research Materials
Miscellaneous