Coat Protein Mutations That Alter the Flux of Morphogenetic Intermediates through the ϕX174 Early Assembly Pathway
- PMID: 28978706
- PMCID: PMC5709602
- DOI: 10.1128/JVI.01384-17
Coat Protein Mutations That Alter the Flux of Morphogenetic Intermediates through the ϕX174 Early Assembly Pathway
Abstract
Two scaffolding proteins orchestrate ϕX174 morphogenesis. The internal scaffolding protein B mediates the formation of pentameric assembly intermediates, whereas the external scaffolding protein D organizes 12 of these intermediates into procapsids. Aromatic amino acid side chains mediate most coat-internal scaffolding protein interactions. One residue in the internal scaffolding protein and three in the coat protein constitute the core of the B protein binding cleft. The three coat gene codons were randomized separately to ascertain the chemical requirements of the encoded amino acids and the morphogenetic consequences of mutation. The resulting mutants exhibited a wide range of recessive phenotypes, which could generally be explained within a structural context. Mutants with phenylalanine, tyrosine, and methionine substitutions were phenotypically indistinguishable from the wild type. However, tryptophan substitutions were detrimental at two sites. Charged residues were poorly tolerated, conferring extreme temperature-sensitive and lethal phenotypes. Eighteen lethal and conditional lethal mutants were genetically and biochemically characterized. The primary defect associated with the missense substitutions ranged from inefficient internal scaffolding protein B binding to faulty procapsid elongation reactions mediated by external scaffolding protein D. Elevating B protein concentrations above wild-type levels via exogenous, cloned-gene expression compensated for inefficient B protein binding, as did suppressing mutations within gene B. Similarly, elevating D protein concentrations above wild-type levels or compensatory mutations within gene D suppressed faulty elongation. Some of the parental mutations were pleiotropic, affecting multiple morphogenetic reactions. This progressively reduced the flux of intermediates through the pathway. Accordingly, multiple mechanisms, which may be unrelated, could restore viability.IMPORTANCE Genetic analyses have been instrumental in deciphering the temporal events of many biochemical pathways. However, pleiotropic effects can complicate analyses. Vis-à-vis virion morphogenesis, an improper protein-protein interaction within an early assembly intermediate can influence the efficiency of all subsequent reactions. Consequently, the flux of assembly intermediates cumulatively decreases as the pathway progresses. During morphogenesis, ϕX174 coat protein participates in at least four well-defined reactions, each one characterized by an interaction with a scaffolding or structural protein. In this study, genetic analyses, biochemical characterizations, and physiological assays, i.e., elevating the protein levels with which the coat protein interacts, were used to elucidate pleiotropic effects that may alter the flux of intermediates through a morphogenetic pathway.
Keywords: bacteriophage phiX174; coat protein; scaffolding protein; virus assembly.
Copyright © 2017 American Society for Microbiology.
Figures
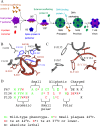
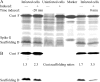
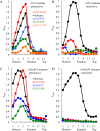
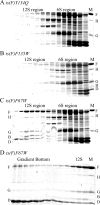
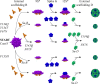
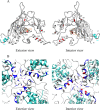
Similar articles
-
Effects of an early conformational switch defect during ϕX174 morphogenesis are belatedly manifested late in the assembly pathway.J Virol. 2013 Mar;87(5):2518-25. doi: 10.1128/JVI.02839-12. Epub 2012 Dec 19. J Virol. 2013. PMID: 23255785 Free PMC article.
-
ϕX174 Procapsid Assembly: Effects of an Inhibitory External Scaffolding Protein and Resistant Coat Proteins In Vitro.J Virol. 2016 Dec 16;91(1):e01878-16. doi: 10.1128/JVI.01878-16. Print 2017 Jan 1. J Virol. 2016. PMID: 27795440 Free PMC article.
-
Identification of an interacting coat-external scaffolding protein domain required for both the initiation of phiX174 procapsid morphogenesis and the completion of DNA packaging.J Virol. 2005 Jun;79(11):6751-6. doi: 10.1128/JVI.79.11.6751-6756.2005. J Virol. 2005. PMID: 15890913 Free PMC article.
-
Building the machines: scaffolding protein functions during bacteriophage morphogenesis.Adv Exp Med Biol. 2012;726:325-50. doi: 10.1007/978-1-4614-0980-9_14. Adv Exp Med Biol. 2012. PMID: 22297520 Review.
-
Filamentous Bacteriophage Proteins and Assembly.Subcell Biochem. 2018;88:261-279. doi: 10.1007/978-981-10-8456-0_12. Subcell Biochem. 2018. PMID: 29900501 Review.
Cited by
-
Disenfranchised DNA: biochemical analysis of mutant øX174 DNA-binding proteins may further elucidate the evolutionary significance of the unessential packaging protein A.J Virol. 2024 Mar 19;98(3):e0182723. doi: 10.1128/jvi.01827-23. Epub 2024 Feb 2. J Virol. 2024. PMID: 38305183 Free PMC article.
-
Low-Temperature Adaptation Targets Genome Packing Reactions in an Icosahedral Single-Stranded DNA Virus.J Virol. 2022 Apr 13;96(7):e0197021. doi: 10.1128/jvi.01970-21. Epub 2022 Mar 14. J Virol. 2022. PMID: 35285686 Free PMC article.
-
Recessive Host Range Mutants and Unsusceptible Cells That Inactivate Virions without Genome Penetration: Ecological and Technical Implications.J Virol. 2019 Jan 17;93(3):e01767-18. doi: 10.1128/JVI.01767-18. Print 2019 Feb 1. J Virol. 2019. PMID: 30429341 Free PMC article.
-
Finally, a Role Befitting Astar: Strongly Conserved, Unessential Microvirus A* Proteins Ensure the Product Fidelity of Packaging Reactions.J Virol. 2020 Jan 6;94(2):e01593-19. doi: 10.1128/JVI.01593-19. Print 2020 Jan 6. J Virol. 2020. PMID: 31666371 Free PMC article.
-
Gut virome of mammals and birds reveals high genetic diversity of the family Microviridae.Virus Evol. 2019 Jun 8;5(1):vez013. doi: 10.1093/ve/vez013. eCollection 2019 Jan. Virus Evol. 2019. PMID: 31191981 Free PMC article.
References
-
- Zlotnick A, Fane BA. 2010. Mechanisms of icosahedral virus assembly, p 180–202. In Agbandje-McKenna M, McKenna R (ed), Structural virology. Royal Society of Chemistry, London, United Kingdom.
Publication types
MeSH terms
Substances
LinkOut - more resources
Full Text Sources
Other Literature Sources