Induction of DNA Damages upon Marek's Disease Virus Infection: Implication in Viral Replication and Pathogenesis
- PMID: 28978699
- PMCID: PMC5709610
- DOI: 10.1128/JVI.01658-17
Induction of DNA Damages upon Marek's Disease Virus Infection: Implication in Viral Replication and Pathogenesis
Abstract
Marek's disease virus (MDV) is a highly contagious alphaherpesvirus that infects chickens and causes a deadly neoplastic disease. We previously demonstrated that MDV infection arrests cells in S phase and that the tegument protein VP22 plays a major role in this process. In addition, expression of VP22 induces double-strand breaks (DSBs) in the cellular DNA, suggesting that DNA damage and the associated cellular response might be favorable for the MDV life cycle. Here, we addressed the role of DNA damage in MDV replication and pathogenesis. We demonstrated that MDV induces DSBs during lytic infection in vitro and in the peripheral blood mononuclear cells of infected animals. Intriguingly, we did not observe DNA damage in latently infected MDV-induced lymphoblastoid cells, while MDV reactivation resulted in the onset of DNA lesions, suggesting that DNA damage and/or the resulting DNA damage response might be required for efficient MDV replication and reactivation. In addition, reactivation was significantly enhanced by the induction of DNA damage using a number of chemicals. Finally, we used recombinant viruses to show that VP22 is required for the induction of DNA damage in vivo and that this likely contributes to viral oncogenesis.IMPORTANCE Marek's disease virus is an oncogenic alphaherpesvirus that causes fatal T-cell lymphomas in chickens. MDV causes substantial losses in the poultry industry and is also used in small-animal models for virus-induced tumor formation. DNA damage not only is implicated in tumor development but also aids in the life cycle of several viruses; however, its role in MDV replication, latency, and reactivation remains elusive. Here, we demonstrate that MDV induces DNA lesions during lytic replication in vitro and in vivo DNA damage was not observed in latently infected cells; however, it was reinitiated during reactivation. Reactivation was significantly enhanced by the induction of DNA damage. Recombinant viruses that lacked the ability to induce DNA damage were defective in their ability to induce tumors, suggesting that DNA damage might also contribute to cellular transformation processes leading to MDV lymphomagenesis.
Keywords: DNA damage; Marek's disease virus; VP22; cell cycle; herpesvirus; oncogenesis; viral replication.
Copyright © 2017 American Society for Microbiology.
Figures
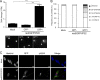
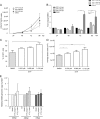
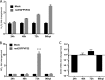
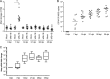
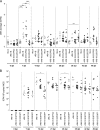
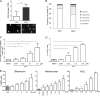
Similar articles
-
The Marek's Disease Virus Unique Gene MDV082 Is Dispensable for Virus Replication but Contributes to a Rapid Disease Onset.J Virol. 2021 Jul 12;95(15):e0013121. doi: 10.1128/JVI.00131-21. Epub 2021 Jul 12. J Virol. 2021. PMID: 34011541 Free PMC article.
-
Marek's Disease Virus Requires Both Copies of the Inverted Repeat Regions for Efficient In Vivo Replication and Pathogenesis.J Virol. 2021 Jan 13;95(3):e01256-20. doi: 10.1128/JVI.01256-20. Print 2021 Jan 13. J Virol. 2021. PMID: 33115875 Free PMC article.
-
Fluorescent tagging of VP22 in N-terminus reveals that VP22 favors Marek's disease virus (MDV) virulence in chickens and allows morphogenesis study in MD tumor cells.Vet Res. 2013 Dec 21;44(1):125. doi: 10.1186/1297-9716-44-125. Vet Res. 2013. PMID: 24359464 Free PMC article.
-
Latency and tumorigenesis in Marek's disease.Avian Dis. 2013 Jun;57(2 Suppl):360-5. doi: 10.1637/10470-121712-Reg.1. Avian Dis. 2013. PMID: 23901747 Review.
-
Telomeres and Telomerase: Role in Marek's Disease Virus Pathogenesis, Integration and Tumorigenesis.Viruses. 2017 Jul 4;9(7):173. doi: 10.3390/v9070173. Viruses. 2017. PMID: 28677643 Free PMC article. Review.
Cited by
-
TOP2β-Dependent Nuclear DNA Damage Shapes Extracellular Growth Factor Responses via Dynamic AKT Phosphorylation to Control Virus Latency.Mol Cell. 2019 May 2;74(3):466-480.e4. doi: 10.1016/j.molcel.2019.02.032. Epub 2019 Mar 28. Mol Cell. 2019. PMID: 30930055 Free PMC article.
-
Hypoxia and HIF-1 Trigger Marek's Disease Virus Reactivation in Lymphoma-Derived Latently Infected T Lymphocytes.J Virol. 2022 Mar 9;96(5):e0142721. doi: 10.1128/JVI.01427-21. Epub 2021 Dec 22. J Virol. 2022. PMID: 34936483 Free PMC article.
-
Mardivirus Infection and Persistence in Feathers of a Chicken Model Harboring a Local Autoimmune Response.Microorganisms. 2020 Oct 20;8(10):1613. doi: 10.3390/microorganisms8101613. Microorganisms. 2020. PMID: 33092272 Free PMC article.
-
The sulphated polysaccharides extract ulvans from Ulva armoricana limits Marek's disease virus dissemination in vitro and promotes viral reactivation in lymphoid cells.BMC Vet Res. 2022 Apr 27;18(1):155. doi: 10.1186/s12917-022-03247-y. BMC Vet Res. 2022. PMID: 35477401 Free PMC article.
-
Marek's Disease Virus Disables the ATR-Chk1 Pathway by Activating STAT3.J Virol. 2019 Apr 17;93(9):e02290-18. doi: 10.1128/JVI.02290-18. Print 2019 May 1. J Virol. 2019. PMID: 30787154 Free PMC article.
References
-
- Arbuckle JH, Medveczky MM, Luka J, Hadley SH, Luegmayr A, Ablashi D, Lund TC, Tolar J, De Meirleir K, Montoya JG, Komaroff AL, Ambros PF, Medveczky PG. 2010. The latent human herpesvirus-6A genome specifically integrates in telomeres of human chromosomes in vivo and in vitro. Proc Natl Acad Sci U S A 107:5563–5568. doi:10.1073/pnas.0913586107. - DOI - PMC - PubMed
MeSH terms
Substances
LinkOut - more resources
Full Text Sources
Other Literature Sources