The chromatin accessibility signature of human immune aging stems from CD8+ T cells
- PMID: 28904110
- PMCID: PMC5626401
- DOI: 10.1084/jem.20170416
The chromatin accessibility signature of human immune aging stems from CD8+ T cells
Abstract
Aging is linked to deficiencies in immune responses and increased systemic inflammation. To unravel the regulatory programs behind these changes, we applied systems immunology approaches and profiled chromatin accessibility and the transcriptome in PBMCs and purified monocytes, B cells, and T cells. Analysis of samples from 77 young and elderly donors revealed a novel and robust aging signature in PBMCs, with simultaneous systematic chromatin closing at promoters and enhancers associated with T cell signaling and a potentially stochastic chromatin opening mostly found at quiescent and repressed sites. Combined analyses of chromatin accessibility and the transcriptome uncovered immune molecules activated/inactivated with aging and identified the silencing of the IL7R gene and the IL-7 signaling pathway genes as potential biomarkers. This signature is borne by memory CD8+ T cells, which exhibited an aging-related loss in binding of NF-κB and STAT factors. Thus, our study provides a unique and comprehensive approach to identifying candidate biomarkers and provides mechanistic insights into aging-associated immunodeficiency.
© 2017 Ucar et al.
Figures
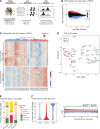
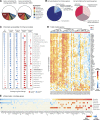
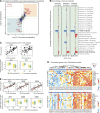
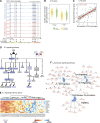
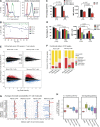
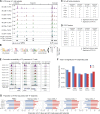
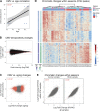
Similar articles
-
Transcriptomic analysis of human IL-7 receptor alpha low and high effector memory CD8+ T cells reveals an age-associated signature linked to influenza vaccine response in older adults.Aging Cell. 2019 Aug;18(4):e12960. doi: 10.1111/acel.12960. Epub 2019 May 1. Aging Cell. 2019. PMID: 31044512 Free PMC article.
-
Elevated IFN-alpha/beta levels in a streptozotocin-induced type I diabetic mouse model promote oxidative stress and mediate depletion of spleen-homing CD8+ T cells by apoptosis through impaired CCL21/CCR7 axis and IL-7/CD127 signaling.Cell Signal. 2015 Oct;27(10):2110-9. doi: 10.1016/j.cellsig.2015.07.005. Epub 2015 Jul 18. Cell Signal. 2015. PMID: 26192098
-
Genome organization and chromatin analysis identify transcriptional downregulation of insulin-like growth factor signaling as a hallmark of aging in developing B cells.Genome Biol. 2018 Sep 5;19(1):126. doi: 10.1186/s13059-018-1489-y. Genome Biol. 2018. PMID: 30180872 Free PMC article.
-
Reduced numbers of IL-7 receptor (CD127) expressing immune cells and IL-7-signaling defects in peripheral blood from patients with breast cancer.Int J Cancer. 2007 Oct 1;121(7):1512-9. doi: 10.1002/ijc.22854. Int J Cancer. 2007. PMID: 17546596
-
Control of chromatin accessibility for V(D)J recombination by interleukin-7.J Leukoc Biol. 2001 Jun;69(6):907-11. J Leukoc Biol. 2001. PMID: 11404375 Review.
Cited by
-
Robust estimation of cancer and immune cell-type proportions from bulk tumor ATAC-Seq data.Elife. 2024 Oct 9;13:RP94833. doi: 10.7554/eLife.94833. Elife. 2024. PMID: 39383060 Free PMC article.
-
An in-depth understanding of the role and mechanisms of T cells in immune organ aging and age-related diseases.Sci China Life Sci. 2024 Sep 2. doi: 10.1007/s11427-024-2695-x. Online ahead of print. Sci China Life Sci. 2024. PMID: 39231902 Review.
-
ImAge quantitates aging and rejuvenation.Nat Aging. 2024 Sep;4(9):1308-1327. doi: 10.1038/s43587-024-00685-1. Epub 2024 Aug 29. Nat Aging. 2024. PMID: 39210148
-
Are immunosenescent T cells really senescent?Aging Cell. 2024 Oct;23(10):e14300. doi: 10.1111/acel.14300. Epub 2024 Aug 7. Aging Cell. 2024. PMID: 39113243 Free PMC article. Review.
-
Repetitive element transcript accumulation is associated with inflammaging in humans.Geroscience. 2024 Dec;46(6):5663-5679. doi: 10.1007/s11357-024-01126-y. Epub 2024 Apr 20. Geroscience. 2024. PMID: 38641753 Free PMC article.
References
-
- Aguirre-Gamboa R., Joosten I., Urbano P.C., van der Molen R.G., van Rijssen E., van Cranenbroek B., Oosting M., Smeekens S., Jaeger M., Zorro M., et al. . 2016. Differential effects of environmental and genetic factors on T and B cell immune traits. Cell Reports. 17:2474–2487. 10.1016/j.celrep.2016.10.053 - DOI - PMC - PubMed
-
- Banchereau R., Hong S., Cantarel B., Baldwin N., Baisch J., Edens M., Cepika A.-M., Acs P., Turner J., and Anguiano E.. 2016. Personalized immunomonitoring uncovers molecular networks that stratify lupus patients. Cell. 165:551–565. (published erratum appears in Cell. 2016. http://dx.doi.org/10.1016/j.cell.2016.05.057) 10.1016/j.cell.2016.03.008 - DOI - PMC - PubMed
MeSH terms
Substances
Grants and funding
LinkOut - more resources
Full Text Sources
Other Literature Sources
Medical
Research Materials