Post-translational palmitoylation controls the voltage gating and lipid raft association of the CALHM1 channel
- PMID: 28734079
- PMCID: PMC5599488
- DOI: 10.1113/JP274164
Post-translational palmitoylation controls the voltage gating and lipid raft association of the CALHM1 channel
Abstract
Key points: Calcium homeostasis modulator 1 (CALHM1), a new voltage-gated ATP- and Ca2+ -permeable channel, plays important physiological roles in taste perception and memory formation. Regulatory mechanisms of CALHM1 remain unexplored, although the biophysical disparity between CALHM1 gating in vivo and in vitro suggests that there are undiscovered regulatory mechanisms. Here we report that CALHM1 gating and association with lipid microdomains are post-translationally regulated through the process of protein S-palmitoylation, a reversible attachment of palmitate to cysteine residues. Our data also establish cysteine residues and enzymes responsible for CALHM1 palmitoylation. CALHM1 regulation by palmitoylation provides new mechanistic insights into fine-tuning of CALHM1 gating in vivo and suggests a potential layer of regulation in taste and memory.
Abstract: Emerging roles of CALHM1, a recently discovered voltage-gated ion channel, include purinergic neurotransmission of tastes in taste buds and memory formation in the brain, highlighting its physiological importance. However, the regulatory mechanisms of the CALHM1 channel remain entirely unexplored, hindering full understanding of its contribution in vivo. The different gating properties of CALHM1 in vivo and in vitro suggest undiscovered regulatory mechanisms. Here, in searching for post-translational regulatory mechanisms, we discovered the regulation of CALHM1 gating and association with lipid microdomains via protein S-palmitoylation, the only reversible lipid modification of proteins on cysteine residues. CALHM1 is palmitoylated at two intracellular cysteines located in the juxtamembrane regions of the third and fourth transmembrane domains. Enzymes that catalyse CALHM1 palmitoylation were identified by screening 23 members of the DHHC protein acyltransferase family. Epitope tagging of endogenous CALHM1 proteins in mice revealed that CALHM1 is basally palmitoylated in taste buds in vivo. Functionally, palmitoylation downregulates CALHM1 without effects on its synthesis, degradation and cell surface expression. Mutation of the palmitoylation sites has a profound impact on CALHM1 gating, shifting the conductance-voltage relationship to more negative voltages and accelerating the activation kinetics. The same mutation also reduces CALHM1 association with detergent-resistant membranes. Our results comprehensively uncover a post-translational regulation of the voltage-dependent gating of CALHM1 by palmitoylation.
Keywords: ion channel; palmitoylation; taste.
© 2017 The Authors. The Journal of Physiology © 2017 The Physiological Society.
Figures
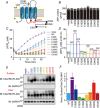
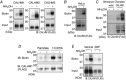
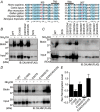
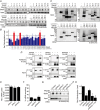
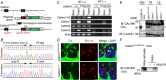
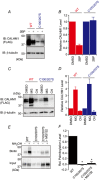
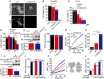

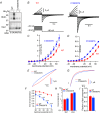
Similar articles
-
Posttranslational regulation of CALHM1/3 channel: N-linked glycosylation and S-palmitoylation.FASEB J. 2021 May;35(5):e21527. doi: 10.1096/fj.202002632R. FASEB J. 2021. PMID: 33788965
-
CALHM1 ion channel mediates purinergic neurotransmission of sweet, bitter and umami tastes.Nature. 2013 Mar 14;495(7440):223-6. doi: 10.1038/nature11906. Epub 2013 Mar 6. Nature. 2013. PMID: 23467090 Free PMC article.
-
Calcium homeostasis modulator (CALHM) ion channels.Pflugers Arch. 2016 Mar;468(3):395-403. doi: 10.1007/s00424-015-1757-6. Epub 2015 Nov 25. Pflugers Arch. 2016. PMID: 26603282 Free PMC article. Review.
-
CALHM3 Is Essential for Rapid Ion Channel-Mediated Purinergic Neurotransmission of GPCR-Mediated Tastes.Neuron. 2018 May 2;98(3):547-561.e10. doi: 10.1016/j.neuron.2018.03.043. Epub 2018 Apr 19. Neuron. 2018. PMID: 29681531 Free PMC article.
-
Ion channel regulation by protein palmitoylation.J Biol Chem. 2011 Mar 18;286(11):8709-16. doi: 10.1074/jbc.R110.210005. Epub 2011 Jan 7. J Biol Chem. 2011. PMID: 21216969 Free PMC article. Review.
Cited by
-
Taste transduction and channel synapses in taste buds.Pflugers Arch. 2021 Jan;473(1):3-13. doi: 10.1007/s00424-020-02464-4. Epub 2020 Sep 16. Pflugers Arch. 2021. PMID: 32936320 Free PMC article. Review.
-
S-acylation of Ca2+ transport proteins: molecular basis and functional consequences.Biochem Soc Trans. 2024 Feb 28;52(1):407-421. doi: 10.1042/BST20230818. Biochem Soc Trans. 2024. PMID: 38348884 Free PMC article. Review.
-
Innate and acquired tolerance to bitter stimuli in mice.PLoS One. 2018 Dec 31;13(12):e0210032. doi: 10.1371/journal.pone.0210032. eCollection 2018. PLoS One. 2018. PMID: 30596779 Free PMC article.
-
ATP Release Channels.Int J Mol Sci. 2018 Mar 11;19(3):808. doi: 10.3390/ijms19030808. Int J Mol Sci. 2018. PMID: 29534490 Free PMC article. Review.
-
Control of astrocytic Ca2+ signaling by nitric oxide-dependent S-nitrosylation of Ca2+ homeostasis modulator 1 channels.Biol Res. 2024 Apr 30;57(1):19. doi: 10.1186/s40659-024-00503-3. Biol Res. 2024. PMID: 38689353 Free PMC article.
References
-
- Bischoff R & Schluter H (2012). Amino acids: chemistry, functionality and selected non‐enzymatic post‐translational modifications. J Proteomics 75, 2275–2296. - PubMed
Publication types
MeSH terms
Substances
LinkOut - more resources
Full Text Sources
Other Literature Sources
Molecular Biology Databases
Miscellaneous