Multicellular Transcriptional Analysis of Mammalian Heart Regeneration
- PMID: 28733351
- PMCID: PMC5598916
- DOI: 10.1161/CIRCULATIONAHA.117.028252
Multicellular Transcriptional Analysis of Mammalian Heart Regeneration
Abstract
Background: The inability of the adult mammalian heart to regenerate following injury represents a major barrier in cardiovascular medicine. In contrast, the neonatal mammalian heart retains a transient capacity for regeneration, which is lost shortly after birth. Defining the molecular mechanisms that govern regenerative capacity in the neonatal period remains a central goal in cardiac biology. Here, we assemble a transcriptomic framework of multiple cardiac cell populations during postnatal development and following injury, which enables comparative analyses of the regenerative (neonatal) versus nonregenerative (adult) state for the first time.
Methods: Cardiomyocytes, fibroblasts, leukocytes, and endothelial cells from infarcted and noninfarcted neonatal (P1) and adult (P56) mouse hearts were isolated by enzymatic dissociation and fluorescence-activated cell sorting at day 3 following surgery. RNA sequencing was performed on these cell populations to generate the transcriptome of the major cardiac cell populations during cardiac development, repair, and regeneration. To complement our transcriptomic data, we also surveyed the epigenetic landscape of cardiomyocytes during postnatal maturation by performing deep sequencing of accessible chromatin regions by using the Assay for Transposase-Accessible Chromatin from purified mouse cardiomyocyte nuclei (P1, P14, and P56).
Results: Profiling of cardiomyocyte and nonmyocyte transcriptional programs uncovered several injury-responsive genes across regenerative and nonregenerative time points. However, the majority of transcriptional changes in all cardiac cell types resulted from developmental maturation from neonatal stages to adulthood rather than activation of a distinct regeneration-specific gene program. Furthermore, adult leukocytes and fibroblasts were characterized by the expression of a proliferative gene expression network following infarction, which mirrored the neonatal state. In contrast, cardiomyocytes failed to reactivate the neonatal proliferative network following infarction, which was associated with loss of chromatin accessibility around cell cycle genes during postnatal maturation.
Conclusions: This work provides a comprehensive framework and transcriptional resource of multiple cardiac cell populations during cardiac development, repair, and regeneration. Our findings define a regulatory program underpinning the neonatal regenerative state and identify alterations in the chromatin landscape that could limit reinduction of the regenerative program in adult cardiomyocytes.
Keywords: ATAC-seq; cell proliferation; epigenomics; muscle cells; myocardial infarction; regeneration; transcriptional profiling.
© 2017 The Authors.
Figures
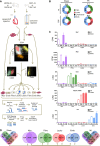
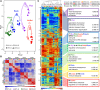
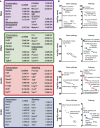
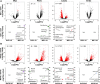
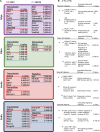
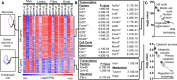
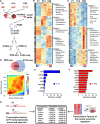
Similar articles
-
Cell-Type-Specific Gene Regulatory Networks Underlying Murine Neonatal Heart Regeneration at Single-Cell Resolution.Cell Rep. 2020 Dec 8;33(10):108472. doi: 10.1016/j.celrep.2020.108472. Cell Rep. 2020. PMID: 33296652 Free PMC article.
-
Regenerative cross talk between cardiac cells and macrophages.Am J Physiol Heart Circ Physiol. 2021 Jun 1;320(6):H2211-H2221. doi: 10.1152/ajpheart.00056.2021. Epub 2021 Mar 26. Am J Physiol Heart Circ Physiol. 2021. PMID: 33769920 Free PMC article.
-
Dynamic Transcriptional Responses to Injury of Regenerative and Non-regenerative Cardiomyocytes Revealed by Single-Nucleus RNA Sequencing.Dev Cell. 2020 Apr 6;53(1):102-116.e8. doi: 10.1016/j.devcel.2020.02.019. Epub 2020 Mar 26. Dev Cell. 2020. PMID: 32220304 Free PMC article.
-
Resetting the epigenome for heart regeneration.Semin Cell Dev Biol. 2016 Oct;58:2-13. doi: 10.1016/j.semcdb.2015.12.021. Epub 2016 Jan 7. Semin Cell Dev Biol. 2016. PMID: 26773213 Review.
-
Neonatal injury models: integral tools to decipher the molecular basis of cardiac regeneration.Basic Res Cardiol. 2022 May 3;117(1):26. doi: 10.1007/s00395-022-00931-w. Basic Res Cardiol. 2022. PMID: 35503383 Free PMC article. Review.
Cited by
-
Generation, functional analysis and applications of isogenic three-dimensional self-aggregating cardiac microtissues from human pluripotent stem cells.Nat Protoc. 2021 Apr;16(4):2213-2256. doi: 10.1038/s41596-021-00497-2. Epub 2021 Mar 26. Nat Protoc. 2021. PMID: 33772245 Free PMC article.
-
Vegfc/d-dependent regulation of the lymphatic vasculature during cardiac regeneration is influenced by injury context.NPJ Regen Med. 2019 Aug 22;4:18. doi: 10.1038/s41536-019-0079-2. eCollection 2019. NPJ Regen Med. 2019. PMID: 31452940 Free PMC article.
-
Developmental programming: adverse sexually dimorphic transcriptional programming of gestational testosterone excess in cardiac left ventricle of fetal sheep.Sci Rep. 2023 Feb 15;13(1):2682. doi: 10.1038/s41598-023-29212-9. Sci Rep. 2023. PMID: 36792653 Free PMC article.
-
Volume Overload Initiates an Immune Response in the Right Ventricle at the Neonatal Stage.Front Cardiovasc Med. 2021 Nov 16;8:772336. doi: 10.3389/fcvm.2021.772336. eCollection 2021. Front Cardiovasc Med. 2021. PMID: 34869688 Free PMC article.
-
Neutrophil-Derived S100A8/A9 Amplify Granulopoiesis After Myocardial Infarction.Circulation. 2020 Mar 31;141(13):1080-1094. doi: 10.1161/CIRCULATIONAHA.119.043833. Epub 2020 Jan 16. Circulation. 2020. PMID: 31941367 Free PMC article.
References
MeSH terms
Substances
LinkOut - more resources
Full Text Sources
Other Literature Sources
Molecular Biology Databases
Research Materials